Planetary Radio • Sep 06, 2023
Subsurface oceans: The hidden potential of Earth-like exoplanets
On This Episode
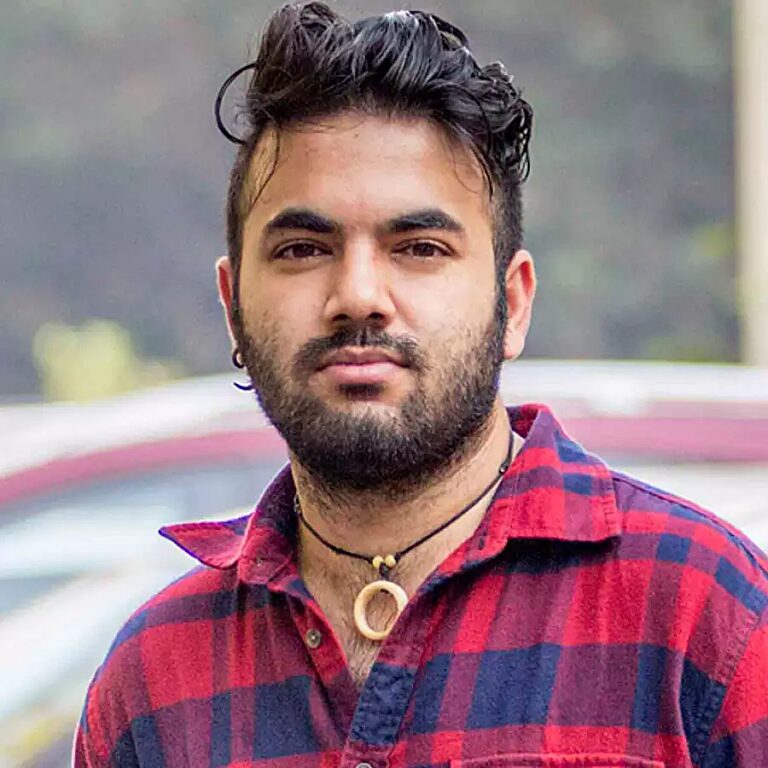
Lujendra Ojha
Assistant Professor for Rutgers University
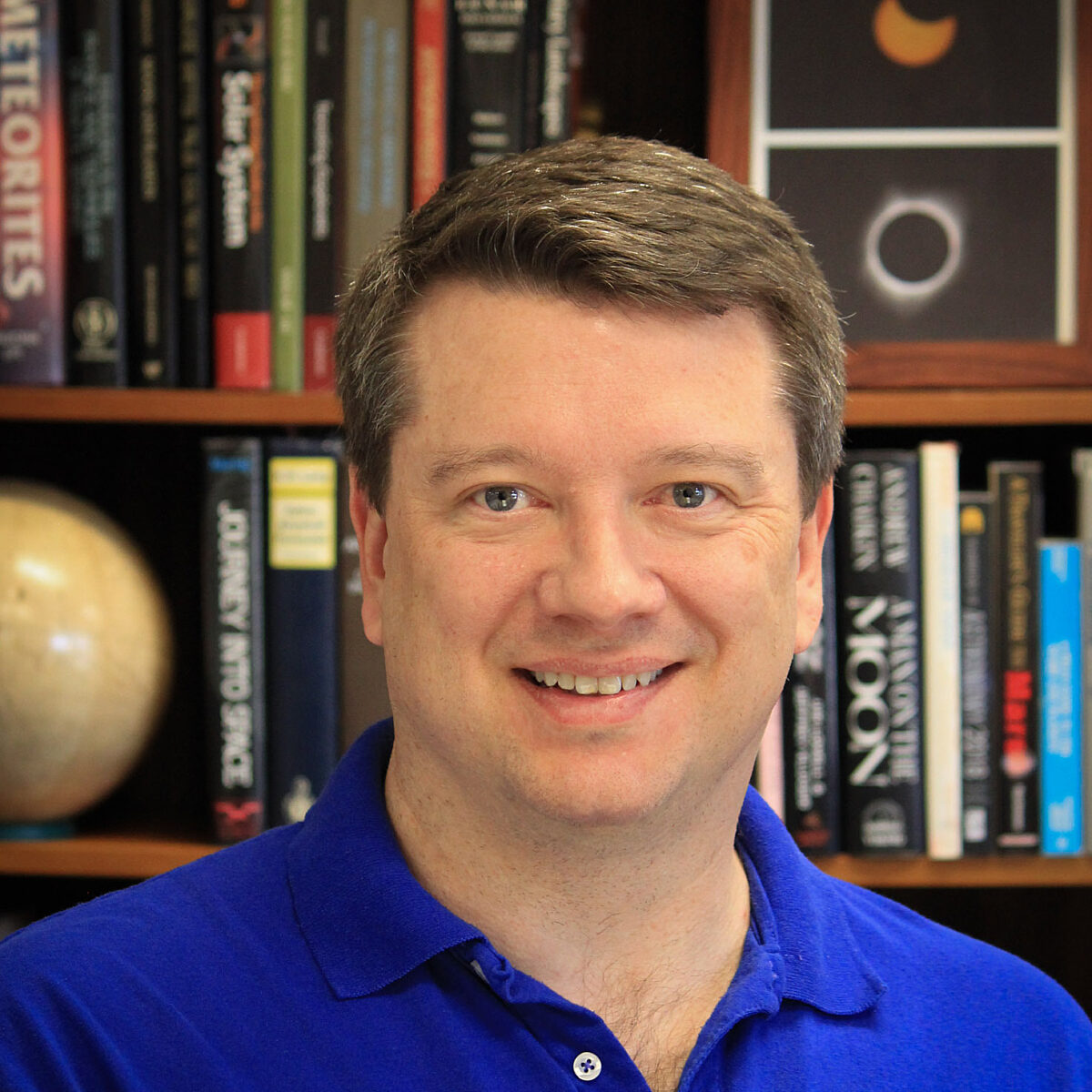
Bruce Betts
Chief Scientist / LightSail Program Manager for The Planetary Society
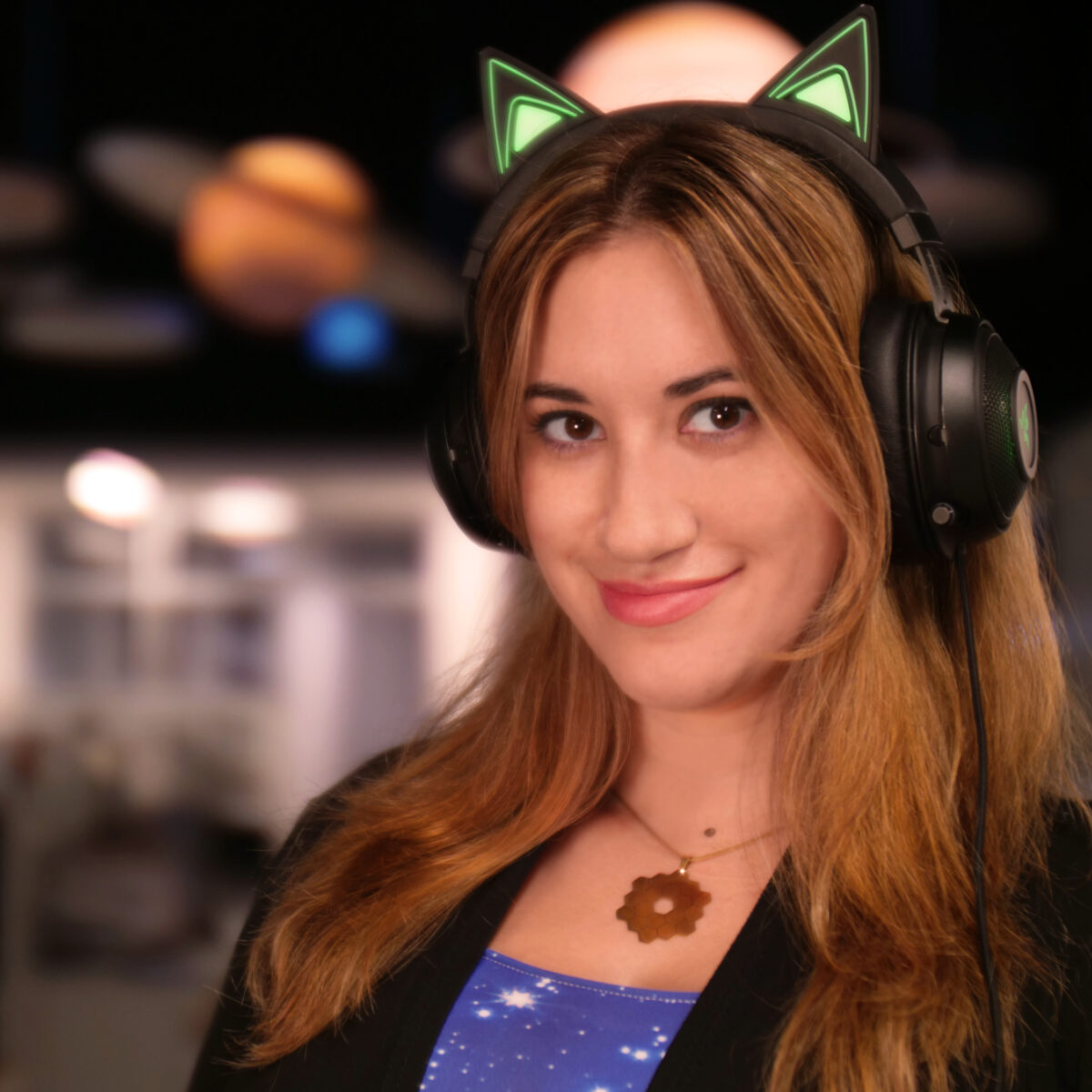
Sarah Al-Ahmed
Planetary Radio Host and Producer for The Planetary Society
Lujendra Ojha, assistant professor at Rutgers University, joins Planetary Radio to discuss how subsurface liquid water on exoplanets orbiting red dwarf stars could increase the likelihood of finding habitable worlds beyond our Solar System. Then we check in with Bruce Betts, chief scientist of The Planetary Society, for What's Up.



Related Links
- Liquid water on cold exo-Earths via basal melting of ice sheets
- Where are the ocean worlds in our solar system?
- What Is the Habitable Zone?
- The Planetary Report March Equinox 2022: Ocean Worlds
- Enceladus, Saturn’s moon with a hidden ocean
- Make your gift to LightSail’s Legacy
- Europa Clipper, a mission to Jupiter's icy moon
- Juice, exploring Jupiter’s icy moons
- The night sky
- The Downlink
Say Hello
We love to hear from our listeners. You can contact the Planetary Radio crew anytime via email at [email protected].
Transcript
Sarah Al-Ahmed: Unveiling Hidden Oceans on distant exoplanets. This week on Planetary Radio, I'm Sarah Al-Ahmed of The Planetary Society, with more of the human adventure across our solar system and beyond. In today's episode, we dive into new research that could reshape our understanding of water and its potential for sustaining life across the cosmos. Lujendra Ojha, assistant professor at Rutgers University joins us to discuss how subsurface liquid water on exoplanets could exponentially increase our likelihood of finding habitable worlds beyond our solar system. Then we'll check in with Bruce Betts, chief scientist of The Planetary Society for What's UP? If you love Planetary Radio and want to stay informed about the latest space discoveries, make sure you hit that subscribe button on your favorite podcasting platform. By subscribing, you'll never miss an episode filled with new and awe-inspiring ways to know the cosmos and our place within it. Now, let's get into the heart of today's episode, my interview with Dr. Lujendra Ojha or Luju. He's a planetary scientist and an assistant professor in the Department of Earth and Planetary Sciences at Rutgers University in New Jersey, USA. His team's recent study presented at the Gold Schmidt Geochemistry Conference challenges conventional wisdom by dramatically increasing the odds of discovering liquid water on distant exoplanets. His work suggests that even worlds with inhospitable surface conditions could harbor subsurface oceans. That's consistent with what we see inside of our solar system. Luju reveals the remarkable mechanisms that could sustain liquid water beneath frozen exteriors and explains how moons like Europa and Enceladus offer compelling examples of such hidden oceans. By investigating planets orbiting M dwarf stars, also called red dwarf stars, the most common type of star in our galaxy, his team's new model suggests that the prevalence of exoplanets with liquid water could be as much as 100 times higher than previously thought. If this is the case, it opens doors to new possibilities for life beyond earth. Let's learn more. It's great to meet you Luju.
Lujendra Ojha: Yeah, great to meet you, and thanks for having me here. Really excited about this.
Sarah Al-Ahmed: I know this isn't the topic of our conversation today, but I remember several years back reading the research about the brine flows on Mars. I ended up giving entire talks and writing articles about that, so it's really cool to meet the person who identified those in pictures originally. That's such cool research.
Lujendra Ojha: Yeah, it was, well, now, let's see, more than 10 years ago when I was an undergrad, and I haven't worked on those features in a long time, but yeah, they're very perplexing. I mean, we still have no idea what they might be, so yeah, it's very perplexing. But 10 years after, we still don't have any answers. But yeah.
Sarah Al-Ahmed: Still cool to have more indications of water on Mars. And as your research shows, there's water all over this universe on all these different worlds and trying to learn more about it could have a huge bearing on our search for life in the universe. So I guess that's the through line of a lot of your research, hunting the water.
Lujendra Ojha: Yeah, I think, for example, I teach this class called Moons and Planets, and I teach another class called Planet Mars, and in each of those class I have about 100 students, so it's a pretty big class. And whenever I mentioned the idea of water and Mars, everyone's excited. But I think it's a weird thing. I mean, if you think about just the water aspect, water is almost everywhere. What's critical for habitability and what I have tried to understand a little bit more is what are the conditions that could create liquid water, which is substantially much harder to get. Water itself, I mean, it's everywhere, so it's not that interesting, but how you create liquid water in some exotic fashion, exotic condition in an exotic planet. I think that's where, I don't know, a lot of my time has been spent.
Sarah Al-Ahmed: I myself really wanted to find exoplanets. I started my undergraduate research in exoplanet hunting. So the fact that we're at this stage now where we're trying to calculate the probability of which exoearths might have subsurface oceans, it's such an advanced point from where I expected we would be at this stage
Lujendra Ojha: I mean, yeah, I mean, 15 years ago when I was starting out in astronomy, before I started out in astronomy, I had no idea about exoplanets. I don't even know it was a thing. And here we are talking about exoearths and potentially habitable exoearths. So yeah, I mean, at least for me, 15 years has been just transformational. So yeah. What's your favorite exoplanet? Do you have one?
Sarah Al-Ahmed: Oh, my favorite. It's a hard one to decide, there's so many cool ones out there, but I mean, I guess it's kind of cliche to say at this point, but I'm really looking forward to the JWST results on the TRAPPIST System. Just the fact that there's so many earth-sized worlds in that system is really exciting. What about you?
Lujendra Ojha: Man, every time I gamble I lose a lot. But if I were to gamble for, I don't know, my favorite exoplanet or the exoplanet that I think might be most appealing in terms of habitability, whatever, I would say Proxima Centauri b, it's the closest, and I don't know, we will dig into it later, but at least theoretically, at least from the modeling side of things, it looks very interesting. But I don't know. I think TRAPPIST System is very, very fascinating because I was telling a friend that all the planets of the TRAPPIST System, it's actually inside the Mercury's radius around the sun, so they're very tidally clustered and super close to this star, and so yeah, that's very fascinating. But yeah, I would say TRAPPIST or Proxima.
Sarah Al-Ahmed: It reminds me a bit of the star systems you'll find in video games like No Man's Sky, just one star and just a bunch of planets stacked really close together against that star all visible in the sky together. I mean, how often are you going to get a circumstance like that?
Lujendra Ojha: I mean, I think there's a lot of interesting science that could also come out of these planets being so clustered together. A friend of mine long time ago was working on this idea of maybe their magnetospheres can be aligned every once in a while when all the seven planets are aligned to each other. And I was like, yeah, these planets do have magnetosphere, maybe... So anyways, so yeah, there's so many fascinating things you can think about, the theoretical scenarios at least, about stuff occurring in TRAPPIST System.
Sarah Al-Ahmed: I'm really glad that I found your paper because I think it was only a few years back I read an article that made this announcement that maybe one in 100 rocky worlds that were orbiting red door stars could have oceans on their surfaces, could be earth-like. And I remember reading that and being like, that sounds a little low to me. What about the subsurface oceans? So your paper made me feel really vindicated.
Lujendra Ojha: It was surprising to me, actually, maybe not surprising, maybe I do understand, but the notion of a habitable zone on exoplanet study has mostly focused on the atmospheric side of things. So normally what we do, my undergrad students for example, do this, I mean you have temperature of the sun, you know how far the planet is, you make some assumption about how bright or how dark this planet might be. So darker things tend to absorb more light. Lighter things tend to reflect more light. So kind of like wearing a black shirt versus white shirt, that's called albedo. So anyway, so you assume some parameters and you can estimate the temperature on these planets. That does not account for greenhouse gases. For example, if you were to do this for Earth, the temperature of the earth comes out to I think like a -10, -30 degrees Celsius, something like that. The point being that if you did not count for atmosphere, earth would be freezing. But we do have greenhouse gases, so that makes things a little bit better. And here we are surviving, we have liquid water. But most exoplanet study in terms of specifically the idea of liquid water revolves around this notion of how far it is from the sun. But if you look at our own solar system, and if you were to ask maybe do a poll of 1000 astro biologists, I would bet my money, again, that a lot more of the astro biologists would say that Mars probably isn't the most appealing place, at least currently, but instead places like Enceladus and Europa might be the most appealing places. And on those places you do have subsurface ocean. And there's a lot of reason to believe that similar situation might have occurred on earth because Earth actually went through several glaciations, snowball earth, which is basically the entire earth was frozen into solid ice, and yet life survived through such events. So the idea of subsurface ocean just was so interesting to me, but I saw very little had been done and discussed in that notion so we thought it's something worthy to explore.
Sarah Al-Ahmed: If we do change this assumption and take into account these icy earth that might have subsurface oceans, how dramatically does that change our understanding of how many worlds might have water?
Lujendra Ojha: I forget who it was. There was a famous physicist who said the real way of doing science is by experiment and replicating and replicating all this theory and models. They're great, but that's just poetry. So I think I'm delving a little bit more into poetry here than maybe bonafide science with empirical evidence, but I think the most important thing that we show in the paper is that if any of these exoearths, Proxima Centauri b, TRAPPIST System, Gza or LHS 1140 b, name your favorite earth-like exoplanet, if these planets were to have water, what we show is that more likely that there is some sort of subsurface ocean than not. So if you were giving two hypothesis, one says this planet has, let's say more water than earth, but it's very cold and there's no water. And then the other hypothesis says this planet has just as much water as earth or even more, but does have subsurface ocean. The second hypothesis, which is that if any earth-like planet has sufficient amount of water, it is more likely that that planet will have liquid water, subsurface ocean than the prior hypothesis, the first hypothesis which says there should be no subsurface water. So what we're dealing is strictly with the notion of can you create liquid water when you have sufficient amount of water there? And so the question I think you were asking is what are the chances of some of these planets having water? I think that's a little bit different question because you're talking about the chemicals themselves. H2O, is that present on some planet? And there's very good reasons to think that a lot of these M dwarf orbiting exoearth might have that because they're in the right place, especially in the night side of these planets are relatively cold. So that should be a great place to trap some cold ice, like cold trapping like we see in the south pole of the moon. So I think there should be water, but we don't make claims about that. What we make claim about in the paper is that if you have ice rich planet, it is very much more likely that that planet would have liquid water than the null hypothesis that there would be no liquid water.
Sarah Al-Ahmed: That's very much reflected in the worlds that we see in our own solar system. I mean, we have one earth with liquid water on the surface, but we have many ocean worlds, all of which have subsurface oceans. So I think you're onto something here. But I did want to ask, why does your paper focus primarily on M dwarf stars?
Lujendra Ojha: This is really fascinating to me because M dwarfs make up about 70% of the stars in our galaxy. And despite that, if one of your listeners, for example, is driving at night right now, you can look out, we can't really see M dwarf, right? They're very small. So with naked eye, you can't really see, they're very small, but yet they make up about 70% of the stars in our galaxy. So that's a big number. If you have a rough estimate about how many stars there are in our galaxy, it's a huge number. I think I ran this number and it comes out to about 24 billion M dwarfs in our galaxy, something like that. And there has been a couple of different studies, and what they did is a survey saying that, all right, we looked at 100 M dwarfs so far, we found earth-like exoplanets in some percent of them. So now let's take that rate and extrapolate it to the whole galaxy. And what they found is almost 40% of M dwarf stars have earth-like planets. And what we mean by earth-like in this sense, again is the size or the mass, the radius that's very much like earth. Now, let's take one step further. And now if we look at all the earth-like exoplanets that have been found, which are mostly almost 99.999% of the earth-like exoplanets that we've found so far revolve around M dwarf. And then out of those, if we say, all right, how much of those planets are very much earth-like in terms of surface temperature, so a basically habitable exoearth, again, all the exoplanets that we've found so far are around M dwarf. It's just a probability study, but I mean if you take what we have so far, our constraints, then what we find is that there's probably a lot of M dwarfs in the galaxy, and turns out many M dwarfs have earth-like exoplanets that are just in that right sort of goldilock zone. So if you just put all these things together, it turns out that there might be many "habitable planets" in the galaxy in M dwarf orbiting systems. So I think it's really fascinating and that's the reason why we focused on M dwarfs.
Sarah Al-Ahmed: The interesting thing about these types of stars is that despite being smaller than our sun, I think of them as very feisty. They're very active, very flaring in their younger years. And that for me always made it an interesting question of how that could impact the habitability of these worlds because even though we're finding these exoearths or earth sized worlds around these M dwarf stars, maybe they're not the best place because they just get bathed in radiation from their stars. But if you then add this extra component of a level of ice protecting those oceans, that could impact the habitability and make them even more favorable for life, potentially,
Lujendra Ojha: Yeah, stars have this main sequence life where they spend a lot of their time in the pre-main sequence phase for M dwarf. There's higher amount of radiation or what we call luminosity coming out in the x-rays and UVs. The habitability of planets around M dwarf, it's such a debated topic, there are so many review papers about this. If there are any students interested in this, or I don't know, whoever is interested in this, they can find a lot of papers on the debate about habitability of planets around M dwarf. But you're absolutely right that early on in their lifetime, the first couple of billion years, there's much more radiation coming out in x-rays and UVs, and especially in let's say you have a planet that does not have a magnetic field, the potential for atmospheric stripping by these intense radiation can be very large. That's where maybe ice does come into play a little bit because a lot of these planets are also tightly locked around the M dwarf stars. And so forget about the day side. So the day side is getting hit with x-rays and UVs and the pre-main sequence lifetime all the time. That's fine. But even in that sense, like you said, there is maybe a couple of meters of ice, maybe a couple of kilometers of ice, maybe tens of kilometers of ice, and underneath those ice there could be subglacial water, and that's a nice little refuge for potential habitability. But now if we look at the night side, the night side, the UV bombardment is a little bit less on the night side, and that night side is much more protected from these intense stellar parameters. At the same time, there could be this notion of basal melting ice melting into liquid water by the planet's own heat that might be going on. And so you might have two very different refuge for potential biota. That's going too far, but again, you're absolutely right that having ice, I mean this is why we are going to Europa, I mean Europa and Enceladus, they both gain a ton of radiation from their planet, Jupiter's intense magnetic fuel and Saturn's intense magnetic fuel, and yet underneath that thick ice cap, it could just be happy, hakuna matata, everyone's happy. For that reason, I think planets are on M dwarf, I mean, it's very appealing to study this idea of subsurface ocean.
Sarah Al-Ahmed: And you touched on this a little bit earlier, but if we were looking to try to find actually earth-like world around these M dwarfs with liquid water on the surface and a nice atmosphere, you would have to have just a kind of ridiculous greenhouse effect in order to establish that around this type of star, right?
Lujendra Ojha: Depending on the distance. So we have this nice chart on the paper where we plot the known equilibrium temperature. So equilibrium temperature is just balancing the energy in from M dwarf, energy radiating out of the planet, and that does not account for greenhouse gas. So there are some planets that actually have equilibrium temperature right around the melting point of water. But then there are some planets like TRAPPIST-1g, I want to say, which has an equilibrium temperature of 200 Kelvin, that's -73 degree Celsius, or 73 below zero, I don't know what that is in Fahrenheit. But yeah, so if you talk about potentially there being liquid water on the surface of TRAPPIST-1g, I mean you would need to augment the temperature by 73 degrees Celsius, so you would need some intense, intense greenhouse warming. I'm not even sure if that's possible. But yeah, I mean you're absolutely right. And I think this is very interesting because this is not just a weird esoteric topic we think about in terms of exoplanets. If you look at our own solar system, about 4 billion years ago, our sun was 30% less brighter than it is today. And 4 billion years ago, yet when we look on earth, when we look at the rock record and when we go to Mars and look at the surface feature, we find plenty of evidence for liquid water. And so this dichotomy or this paradox between the astronomical stellar evolution models that tells us that sun was much, much, much less bright 4 billion years ago. Yet when you look at the geology, you find water on both of these planets creates this paradox called the faint young Sun paradox. The problem is a little bit much more intense for Mars because Mars is, what? 43 million miles farther away than Earth is. Mars would've received much less solar radiation. So how do you solve that faint young Sun paradox? So going back to your original query about you needing greenhouse gases. On Mars, we can experiment with all kinds of different greenhouse gases and it's not that easy, right? So there's lots of debate about what the gases could be, or if at all, most of the features we see was caused by surface runoff or surface liquid water. There's also a competing view that most of the features that indicates liquid water on Mars could have been formed by similar idea of basal melting, that Mars was totally covered by thick ice caps 4 billion years ago everywhere, and these river like networks we see called valley networks could have been formed by subglacial melting and subglacial drainage. So we don't really know. It's an active topic of debate and active topic that a lot of people are working on, but the point being that we see similar effect 4 billion years ago in our own Solar System, where if it wasn't greenhouse gases, then this idea of basal melting would be very, very important to explain all the liquid water features we see in our own solar system.
Sarah Al-Ahmed: We'll be right back with the rest of my interview with Lujendra Ojha after the short break.
Bill Nye: Greetings, Bill Nye here, CEO of The Planetary Society. Thanks to you, our LightSail program is our greatest shared accomplishment. Our LightSail 2 spacecraft was in space for more than three years, from June, 2019 to November, 2022, and successfully use sunlight to change its orbit around earth. Because of your support, our members demonstrated that highly maneuverable solar sailing is possible. Now it's time for the next chapter in the LightSail's continuing mission, we need to educate the world about the possibilities of solar sailing by sharing the remarkable story of LightSail with scientists, engineers, and space enthusiasts around the world. Now look, every successful Mission has a book to go with it, Hubble, OSIRIS-REx, the Mars Rovers, and now LightSail. We're going to publish a commemorative book for your mission. It will be filled with all the best images captured by LightSail from space, as well as chapters describing the development of the mission, stories from the launch and its technical results to help ensure that this key technology is adopted by future missions. I know I'll need at least one for my coffee table and maybe another one from my bookshelf. Along with the book, we will be doing one of the most important tasks of any project. We'll be disseminating our findings in scientific journals, at conferences and other events, and we'll build a master archive of all the mission data. So every bit of information we've collected will be available to engineers, scientists, and future missions anywhere. In short, there's still a lot to do with LightSail, and that's where you come in. As a member of the LightSail Mission Team, we need your support to secure LightSail's legacy with all of these projects. Visit Planetary.org/Legacy to make your gift today. LightSail is your story, your success, your legacy, and it's making a valuable contribution to the future of solar sailing and space exploration. Your donation will help us continue to share the successful story of LightSail. Thank you.
Sarah Al-Ahmed: Other than surface temperature, what other factors could impact whether or not there's basal melting on these worlds?
Lujendra Ojha: So surface temperature plays a role, but one of the bigger factor that we talk a lot about in the paper is what is called the heat flux or the geothermal heat flux. So if you were to start digging in your backyard, as you go deeper and deeper and deeper into the earth's crust, what you will find is that the temperature increases more and more and more. So that's the gradient, right? So gradient is just a jargon for slope. So that's how geothermal gradient basically tells you how fast the temperature rises as you go deeper and deeper inside a planet. That has a tremendous amount of effect on whether an ice of a given thickness would melt or not. And so on earth, the typical value, the unit don't matter, I'm just going to give you a comparison of Earth, Mars and moon. On earth, typically around the continents, if you go to super hot area like Yellowstone where we know that there is this magma chamber underneath Yellowstone, it might erupt any time. That's what they say, at least. If you were to measure the geothermal heat flux, it is very high there, it's almost 1000 milliwatt per meter square. I live in New York. New York is not that geologically active, that same geothermal gradient in New York is about, I think 40. Okay? So substantially less. If you go in the middle of the ocean and you were to measure geothermal heat gradient right around mid-oceanic ridge, we about 100, 200, something like that. So that's what we typically see on earth. Now, if we go to Moon, the astronauts in the Apollo mission era, they actually did this heat flow experiment, and I think the number is like 15 to about 20 in the Apollo 13 and 15 landing sites. So Moon, as you go deeper and deeper in the moon, it gets substantially less hot than on Earth. On Mars, we don't have direct measurement. InSight lander was trying to measure that, but we didn't succeed. But based on indirect evidence, we think the maximum heat flow on Mars right now is probably about 30. And so Earth because of various different things, it's relatively hot. And so this notion of the planet's own internal heat, which is, it can be caused by a number of different factors, but the main thing that is responsible for that internal heat is radioactive elements like uranium, corium and potassium. They undergo radioactivity, and in the process they're releasing heat. That's the reason why it gets hotter and hotter, right? There's radioactivity, there's other stuff, but radioactivity is the most important thing. And so in this mathematical game of how do you melt an ice slab of some thickness, surface temperature plays a very big role. But then also equally important factor is how hot is that planet? How warm is that planet, not by the sun, but by its own internal heat?
Sarah Al-Ahmed: I think what's really interesting about this is that in our own solar system, we see that most of the ocean worlds are actually moons orbiting larger planets because they have this additional thing that can heat them up, these tidal forces, this tidal interaction that can flex the world as it goes around the planet, and that adds energy to the system. But as you pointed out earlier, a lot of these worlds that are orbiting these M dwarfs are actually tidally locked to their stars. So that means they're going to be getting less of that flexor and just to get the same amount of that tidal force, they'd have to be so close to their star. And they're way bigger, so they're less likely to flex. There's a lot of reasons why they wouldn't have the benefit of this tidal heating, so we're going to have to rely on radioactivity.
Lujendra Ojha: There are some studies that have looked into the evolution of the orbit of these planets, and there are some studies that suggest that before getting tidally locked to the star, that there was actually this tidal forces that could have played a role in generating heat on these planets. So the magnitude of that heating, tidal heating is much stronger. It can be much stronger than the heating from radioactivity alone. So if that was the case, then you go into this sort of scenario, it's called the runaway scenario, I think, and it's basically that you would desiccate the planet because you have so much heat being created by tidal forces that if there was any water, you would just completely evaporate it from the surface. So the surface wouldn't have any liquid water nor any sort of solid ice, and you would completely desiccate the planet. The atmosphere might be completely stripped over time. And so then in that case, you might end up with a planet that resembles something like Venus. If tidal heating is strong, is very strong, then it could lead to complete desertification, completely evaporation, and that you would end up with a planet like Venus.
Sarah Al-Ahmed: But in the scenario that you do have an icy world where you get basal melting, there are a lot of really cool things that you can do with the phase states of water within these worlds. And I think people usually just think about this ice, water vapor, liquid water kind of situation we've set up in early science classes, but there are a lot of cool different types of ices, very pressurized water and things that could be existing in these worlds.
Lujendra Ojha: And this is mostly thanks to one of my collaborators, Baptiste Journaux, who's this great ice physicist guy at University of Washington. But yeah, he's created these amazing dataset and models where we can look at what happens to ice as you apply more and more pressure. And like you said, yeah, it's not just like the ice that's in your coffee. You take that ice and you put it under intense amount of pressure. A lot of the parameters, specifically what we call thermal physical parameters, so density for example, or the ability of the ice to conduct heat, thermal conductivity, all of those things changes with pressure and also temperature. And so think about this exoplanet called LHS 1140 b. That planet's surface gravitational, the gravitational acceleration is about 24 meter per second square. Right? Compare that to earth, which is about 9.8 meter per second square. So let's do a thought experiment. So now let's take, let's say a five kilometer thick ice on earth and put the same five kilometer thick ice on this planet, the super earth LHS 1140 b, what you will find is that at the base of the ice in LHS 1140 b, because of the higher acceleration, the ice would feel much, much, much higher pressure because of its own weight. So weight is mass times gravity. And so because of that, the fundamental transformation with pressure is going to be much more stark and much more profound on LHS 1140 b. At the same point, if we look at some other exoplanet electro TRAPPIST-1g for example, which I think has a little bit less surface gravity than we see in earth, that same five kilometer slab of ice would not be at the same pressure as we see on earth. And so we have to take that into consideration because if you think about ice VI, so this is a really, really pressurized form of ice, it would take you about 360 Kelvin to melt ice VI into liquid water. So let's say underneath this really, really thick ice sheet of an exoplanet, there's ice six. And let's say somehow you were able to create enough... Let's say you lit a fire or you had enough energy to heat up the base of the ice to zero degrees Celsius or a couple degrees Celsius, that ice would not melt because it is very different phase, it is very much pressurized. So you have to be able to provide a source of heat that goes up to 360 degrees Celsius to even melt that ice VI into liquid water. And so that's another thing that you really have to take into consideration. We obviously don't know how thick potential water layer could be on these planets. We have absolutely no idea if there is even solid ice on TRAPPIST-1 System, let alone how thick they could be. And so in modeling studies, you do what's called parameter sweep, so you explore what you might need for a range of parameters. So you say, let's assume that some of these planets have earth-like water, earth-like water mass fraction. How much water could these planets have if it was very earth-like? Now, let's consider a very different scenario where we say that these planets are very water poor. Then what happens? How much heat flow would you need? And is it possible for the basal melting to occur? And on the other flip side, you assume that these planets are even more water rich than earth. So LHS 1140 b, the super earth that I've been talking about, some of the constraints from the astronomy side of things where you have one measurement, which is your density, which you have your mass and you can figure out the potential internal structure. One of the studies, they're constrained on how thick the ice layer could be is about 700 kilometer. And that's a crazy number. I mean 700 kilometer thick ice layer on a planet that has very high surface gravitational acceleration, 24 meter per second square. If that is really true, then we don't even know what ice phase these systems probably could be. We don't have the data. I mean, we weren't able to do any sort of modeling for that sort of system. The highest we could go on LHS 1140 b was 70 kilometer at the maximum pressure. We've done experiments, and so you can get super crazy ice, maybe something that we haven't even thought about yet. But yeah, the modification of ice with pressure, well, it brings a lot of chaos into this simple way of thinking about all ice melting into liquid at water and then evaporating into vapor.
Sarah Al-Ahmed: I had to bring it up because I remember how completely mind blown I was to learn that there were different ices when I was going through undergrad. I'd never been told that before. You always just assume that water freezes, it expands and turns into this one type of ice. No, there are many, many types of water ice, and I encourage people to blow their own minds by looking this up. So we've talked about LHS 1140 b, and I'm so glad you brought it up because I was going to ask you about it, but we also talked a little bit about Proxima Centauri b earlier. Do we have any understanding of what the situation might be on that world?
Lujendra Ojha: Yeah. Proxima Centauri b, the equilibrium temperature, which I was talking about earlier, is I think about 257 kelvin. So that's about what, less than -20 degrees Celsius. And so what we found with Proxima Centauri b is that if it's anywhere like Earth, let's say it has water about the same that we see on earth, then it's so easy to create melt water by basal melting on Proxima Centauri b, just because the surface temperature is so high, it's about 257 Kelvin, and so is TRAPPIST-1e. But there are two things that make it really interesting for Proxima Centauri b. One is, again, like I said, the temperature, even without greenhouse is 257 Kelvin. And so if there is actually greenhouse gases in Proxima Centauri b, I mean, forget about me, forget about everything I said, that planet is probably just in the right place, and it could have liquid water, not bearing all the stellar harsh effects from M dwarf. But if it has any sort of water with greenhouse gas, it might actually be warm enough for surface liquid water. But let's say that is not the case and that it's just at 257 Kelvin. Then the other thing that really helps with Proxima Centauri b is that it's just a little bit bigger than earth. And so that same amount of ice that you would put on Proxima Centauri b gets crushed, gets pressurized just a little bit more than on earth. And the ice that we're used to, the ice we're most used to on earth is called ice phase one. If you start pressurizing that ice phase one, the amount of heat that you need to melt that actually goes lower and lower and lower. So if you take ice phase one, again, like your ice cube and start putting pressure on it, instead of melting at zero degrees Celsius, under sufficient pressure, you can have actually a -20 degrees Celsius, and it will still transform into look at water. So again, this is where the ice phases things come into play a lot. And so with Proxima Centauri b, what's great is that the surface temperature is high enough that it's appealing, but also because of its slightly higher gravity, you don't need 273 Kelvin, zero degrees Celsius to melt some of these thick ice sheets. It can be substantially lower and you will still have liquid water. But that's from the modeling side. We have absolutely no constraint whatsoever on whether there could be 10 kilometer thick ice sheets on Proxima Centauri b, or it's not even possible for a meter of ice to be on these on Proxima Centauri b.
Sarah Al-Ahmed: If there are these scenarios on these worlds where basal melting is creating these subsurface liquid water oceans, how long would these oceans be stable for?
Lujendra Ojha: Yeah, that's another thing that we studied a little bit. It obviously depends on, again, how much heat is being generated by the planet's own internal heat. So let's assume that there is a planet very much like Earth, and it started out with the same amount of these heat producing elements, uranium, corium, potassium as earth did. And so we can do very simple back of the paper, back of the envelope experiment and say, how long could this potentially exist for? And I think we consider two case. One where the planet cools very, very, very fast, which we call the accelerated cooling model. And then in the other, we consider where the planet cools a little bit not as accelerated. And in both case, what we found, so when you consider a slow cooling model, that liquid water created by geothermal heat can survive for about 3 billion years. If you assume a very, very fast cooling of the planet, then maybe about 500 million years. So it's totally up to parameters, these numbers that I'm giving you are totally model dependent. But if you think about, I think a way to think about maybe is to think about the half lives of these elements, uranium, thorium, potassium, their half lives are in billions and tens of billion years. And so as long as you have sufficient amount of these elements, and let's say that there isn't something catastrophic happening, then under normal situation, I think the liquid water created by basal melting should exist for a long period of time.
Sarah Al-Ahmed: And that bodes well for the potential for life out there. But it also, I think this entire understanding of the fact that there's probably more worlds with subsurface oceans than surface oceans like Earth really underscores for me just how lucky we are to live on this planet. Because can you imagine how many worlds might be out there potentially with life, but they'll never be able to look up and see the stars. They might not know the broader universe because they're under this layer of ice. Who even knows what's out there?
Lujendra Ojha: There's this really interesting paper, and it's called On the Likelihood of Life as a Function of Cosmic Time, and it's basically a probability, statistical paper where the authors make the point that if you look at the most habitable planets, according to our current understanding, they seem to be M dwarfs. And unlike the stars that we live in, like our Sun-like stars, for example, M dwarfs can survive for trillions of years, and that has to do with the interior dynamics where most of the heat is transported by convection in M dwarfs, so there isn't this helium buildup. Anyways, the point being that M dwarfs can survive for trillions of years and so these planets around M dwarfs, if it's just by internal heat, could be habitable for lot longer. If you just think about our own earth, I mean, based on our understanding, the Sun will go kaboom in a couple of billion years and we will not survive indefinitely. But in M dwarfs, there's at least potential for, if any life exists, that there is a habitable environment, if that planet is habitable, for a very, very long period of time. And so this paper basically makes a case that we might be a fluke in the sense that we exist so early on in the birth of our universe. I mean, given that the universe is only about 14 billion years, or the fact that we exist might be a fluke, and the best statistically most probable time to find a widespread life in the universe might be much, much later on, that we haven't gotten there yet. But then the other thing about what you said about water world is very fascinating too because there's another study, they looked at the idea of do you need land for technologically advanced civilization to come about? And the answer is, yeah, you do need land, at least based on our experience here on earth, that the technologically intelligent species like humans, we needed land and that's where we created technology. And so the paper makes a point that you can't have too much land. So if you only have 100% land like Venus, then you're not going to have water so you might not even proliferate life, let alone technologically advanced life. But if you have completely just 100% water, then you might not create intelligent life either because we don't have any data to suggest that. And so yeah, it could be that, I mean, again, this is not science, this is my philosophy... well, I guess my poetry, and so no one should take this seriously, but it would be very ironic in the sense that we find worlds after worlds that just have tons of water, but there is no land, and maybe technological intelligence never came about. If you think about spaceship, this is very weird analogy that I was giving someone, but if you think about spaceship, I mean humans, let's say we were to become interstellar species. All we need is air inside that spaceship. Let's say technology or technologically advanced species were to actually even come about underwater, their spaceship would have to be filled with water. Water is 1000 kilogram per meter cubed. That's really, really heavy.
Sarah Al-Ahmed: That's a great point.
Lujendra Ojha: So I don't know. So there's a lot of interesting things to think about like what would a universe completely infested by water world beings look like? But anyways, that's more poetry than science, at least at this point. Yeah.
Sarah Al-Ahmed: So I think the next question for me is how do we find these worlds? Because if they're covered in a layer of ice, how from earth can we look out into the stars, find these worlds, and then make some assumptions about whether or not they have subsurface oceans? We might need all kinds of technological advancements. Is it even possible? Who knows?
Lujendra Ojha: Yeah, and I've thought about this quite a bit. How would you go on about proving something like this might even exist? And I haven't run the numbers yet, but I think one of the things that we could potentially strive to do or try to look for is even before we sent spacecraft to the icy moons, there was at least some sort of prediction that these might be ocean worlds just based on tidal energy that they receive. And then what we saw with Enceladus, and some claim that they've seen this with Europa as well now, is these geysers, so basically plumes of water coming out. So I wondered, is it possible that we might be able to see something like that 100 years from now with a more advanced telescope? If we can increase our nominal resolution, is it possible that we might see time varying amount of water vapor in the atmosphere, which might support the idea of something analogous to the plumes we see in Europa and Enceladus? And so that's a little bit in the future, but now at the present time, I think one thing that we could potentially look for is planets that are very close to the Sun, where the day side should be just bombarded. So the daytime should be so hot that there should be no water. And yet if we were to find water vapor around these planets, then that I think will support the notion that there must be a night side that has thick ice caps where water is being evaporated. Because at least in the day side, it should be very warm, and if my understanding is correct, then we shouldn't really see intense amount of water vapor on these planets. So if we do actually see water vapor around these planets, what we think, what we know that it should be really hot, then that may be implying that there is a night side that has all the ice and the water vapor that you see in the atmosphere is from the evaporation of the ice on the night side. Those are a couple of working hypothesis. It's a relatively difficult question to answer, what we might look for, but I think those two are, at least for right now, working hypothesis.
Sarah Al-Ahmed: Yeah, there's a lot of things that we would have to understand more about, what fraction of worlds with subsurface oceans even develop cracks, especially without all that tidal flexor going on? There's just a lot that we don't understand, but I mean, just the fact that there were worlds at all around other stars was purely speculative even 40 years ago. So this is a great starting point, I think, for the next step in trying to look for habitable worlds. It's a wide and beautiful universe and we've got one of the coolest planets out there, not literally, but yes.
Lujendra Ojha: Thankfully. Yeah.
Sarah Al-Ahmed: Thankfully. Yeah. But thanks for doing this research and for coming on the show to tell us about it. I think this is just really cool, and it fills me with hope, knowing that there's probably so many worlds out there that might have liquid water oceans, and we're just scratching the surface of what it means to be alive and what living creatures need. But to try to find creatures like us out there, this is a great starting point.
Lujendra Ojha: Thank you so much for having me. And yeah, I think it's a very fascinating topic.
Sarah Al-Ahmed: Well, thanks for joining me, Luju.
Lujendra Ojha: Yeah, thank you so much, Sarah.
Sarah Al-Ahmed: The universe is full of surprises. Even seemingly harsh environments can harbor the conditions necessary for life to thrive. We're only just beginning to unravel the mystery of habitability in our universe, but it's a good start. Now let's check in with Bruce Betts, the chief scientist of The Planetary Society for What's Up? Hey, Bruce.
Bruce Betts: Oh, hi. Should we get to something?
Sarah Al-Ahmed: Yeah, let's get to the random space fact. So what's our random space fact this week?
Bruce Betts: The what?
Sarah Al-Ahmed: The...
Bruce Betts: [inaudible]. So if Mercury were traveling at freeway speeds, let's say, just go with this for a moment with me. Mercury's traveling at freeway speeds, then Neptune being chill would be doing a light jog around the sun.
Sarah Al-Ahmed: I was wondering where you were going with that, but that-
Bruce Betts: The point is mercury goes a lot faster and then everything slows down due to that whole balancing forces thing as you go out. So Neptune's kind of doing a light jog, a really, really fast walk and mercury's over there just and making that noise, but we'll have to send atmosphere for it to make that noise.
Sarah Al-Ahmed: If anybody wants to send us artwork of Mercury just cruising down the highway and Neptune doing a light jog, I will cherish it forever.
Bruce Betts: That would be entertaining. I am not capable of doing that, but I look forward to whether someone who is gives us a little something. Yeah, Mercury with its wayfarers on.
Sarah Al-Ahmed: And this was something I was thinking a lot about recently because I was just talking with Luju about all these worlds out there that probably have these subsurface oceans. And I don't know, from where I'm sitting from all the data we're looking at, it looks like probably most of the liquid water in the universe is probably underneath an ice sheet somewhere. And I really do wonder, if life did develop on those worlds, what would they have to go through to actually get through that ice to realize the scope of the universe? That's the thought that keeps me up at night these days after that interview.
Bruce Betts: Wow. Maybe they develop, what do they call it, sawfish, those big saw kind of things and they start sawing through the ice.
Sarah Al-Ahmed: Several kilometers later. Or maybe what I'm thinking is if there's a world that has enough tidal flexor to create some kind of crack that would produce a jet or a plume or something, maybe somewhere out there, there's one crack big enough to let them find their way to the surface and see the stars, if they can survive it, and if they have eyeballs.
Bruce Betts: Wow, that is an interesting and weird thing. Okay, now you've just got me thinking about that. Fortunately, I don't think I'll lose sleep. Hey, big dog. How you doing? Max came to say hi too.
Sarah Al-Ahmed: Hi, Max.
Bruce Betts: All right, everybody go out there, look up the night sky and think about... What's that, Max? Think about that. Thank you and good night.
Sarah Al-Ahmed: We've reached the end of this week's episode of Planetary Radio, but we'll be back next week to discuss how Jupiter's early luminosity impacted the Galilean moons forever. You can help others discover the passion, beauty, and joy of space, science and exploration by leaving a review and rating on platforms like Apple Podcast. Your feedback not only brightens our day, but also helps other curious minds find their place in space through Planetary Radio. You can also send us your space thoughts, questions, and poetry at our email at [email protected]. Or, if you're a Planetary Society member, leave a comment in the Planetary Radio space in our member community app. Planetary Radio is produced by The Planetary Society in Pasadena, California and is made possible by our starry-eyed members. You can join us as we support the search for water worlds at Planetary.org/join. Mark Hilverda and Rae Paoletta are our associate producers. Andrew Lucas is our audio editor. Josh Doyle composed our theme, which is arranged and performed by Pieter Schloser. And until next week, Ad Astra.