Emily Lakdawalla • May 11, 2010
Radar glories in Titan rivers
Wow, this is a cool paper.
Here's the gist: the Cassini RADAR team has spotted some river channels on Titan that shine so brightly in radar images, there must be something special going on to explain that brightness. What we're seeing is the same phenomenon that makes reflective paint on street signs shine brightly in headlights. But instead of tiny plastic beads embedded in reflective paint, we're seeing the internal reflections from pebbles and cobbles of ice, as big as tennis balls or even footballs, lining Titan's river beds.
That's the hypothesis explored in "Radar-bright channels on Titan," a paper just published in Icarus by Alice Le Gall and four coauthors (plus the Cassini RADAR team). I read the paper over lunch, and it was fun: every time I had a question, my question was answered in the very next section of the paper! Le Gall and her coauthors really made a case that convinced me.
The paper is concerned specifically with two river beds observed in the T44 flyby of Titan, when the Cassini RADAR instrument acquired an image swath across the southwestern edge of the bright region on Titan known as Xanadu. Xanadu is a huge area of Titan, 2,500 kilometers across, that's often imagined as a "continent" on Titan, although in truth it's not exactly analogous to Earth continents. Here's part of the T44 swath, shown at half of its full resolution. I grabbed it from Jason Perry's wonderfully useful Titan RADAR SAR Swaths page (thanks again for that resource Jason!)

It may be useful here to give a reminder of how the RADAR instrument gets its images. I won't go into much detail but a key fact is that radar imaging, as I described recently in my post on radio imaging of asteroids, is an active method; it's kind of like shining a flashlight into a dark room. When Cassini took the image swath I posted above, it was flying along the moon, looking slightly sideways, shining its radio signal on the surface, and using that reflected radio signal to build an image of what it saw as it flew past. Things that are bright in radar images are usually bright for one of two reasons: either they are surfaces that are sloping and happen to have slopes that are perpendicular to Cassini's look direction, or they are surfaces that are rough at the same scale as the wavelength of Cassini's radar, which is 2.3 centimeters, which means they have lots of little facets that happen to line up perpendicular to Cassini's look direction. Smooth surfaces, unless they are oriented just right, reflect most of Cassini's radio signal away from the spacecraft, so they look dark in radar images.
The version of the radar swath that is inline in this blog post is VERY low resolution but you can actually make some key observations without clicking to enlarge. Xanadu is all the bright stuff; it has lots of Cassini-facing slopes. On the right half, you can see the edge of Xanadu's hilly terrain, where it is bounded by the dark plains. The dark plains are probably smooth and flat, hence, dark. There are two especially bright squiggly channels on those dark plains; those are the subject of the paper. Here is a closeup view of one of them.

So let's get into the paper. First, Le Gall (by which I mean "Le Gall and her many coauthors") describes the channels. They are the largest radar-bright channels yet observed on Titan; you can see from the scale bar that the one I show here is more than 100 kilometers long, and several kilometers wide in places; it gets narrower as it goes downstream. (The flow direction can be inferred from the angle at which upstream tributaries join the channel and from regional topography.) Both channels have relatively low slope, likely under one degree, and are somewhat sinuous. They appear in an unusual location on Xanadu, where it has an unusually sharp, straight boundary with the darker plains. That boundary has been hypothesized as a possible tectonic fault, where Titan's crust broke and one side slid downward while the other side rose upward.
The channels are also incredibly radar-bright. They are brighter than anything else in the image except for slopes in the Xanadu hills that faced the direction Cassini was gazing from (that's from the top, at an incidence angle of 20 degrees off of vertical). Their brightness can be quantified by measuring the "radar cross section," which is measured from the power of the reflected radar signal and reported in units of decibels or dB. The brightness of these channels is greater than 4 dB, and in some places approaches 5 dB. (For comparison, the brightness of the plains surrounding the channels is around -2 to -3 dB.)
Don't worry about what radar cross section means, or the significance of decibels in this context; the important thing is, as Le Gall goes on to say in the discussion section of the paper, that it's really hard to explain radar cross sections higher than 3 dB for the viewing geometry of Cassini during this flyby for the kinds of materials that are known to occur on Titan's surface -- things like hydrocarbons, water ice with or without ammonia mixed in, and the hydrocarbon-ammonia polymer gunky compounds known as tholins.
What could be enhancing the amount of backscattered radio waves in these two river beds? It can't be the same effect as the one that causes Xanadu's hillslopes to be bright; the entire surface of the 100-plus-kilometer-long river can't be tilted at 20 degrees to horizontal, because then it would have to be a huge mountain, which it is evidently (from topography measurements) not.
So Le Gall starts thinking outside the box. One way you intentionally make enhanced backscatter is to build corner reflectors. A corner reflector is like three walls of a cubical box, with the walls meeting at angles of 90 degrees. No matter what angle you shine a light into such a box, the light rays bounce off the walls and come back at you. But there's really no way to create such corner reflectors inside a river channel naturally.
For Jupiter's moons, some have proposed that little voids or rocks suspended within the surface ice can enhance radar backscatter; if it works on Ganymede, it should also work on Titan. But this mechanism can't make backscatter go as high as 4 or 5 dB under the viewing conditions that Cassini had on this flyby.
With all those ideas eliminated, Le Gall advances a solution: round cobbles of ice. The idea of round cobbles of ice on Titan is not outlandish at all, thanks to that incredibly valuable single image of Titan's surface sent back by the Huygens probe:

Imagine that the river valley happens to be filled with roughly spherical cobbles similar in size to the ones at the Huygens landing site. Here's a diagram showing what would happen to incoming radio waves:
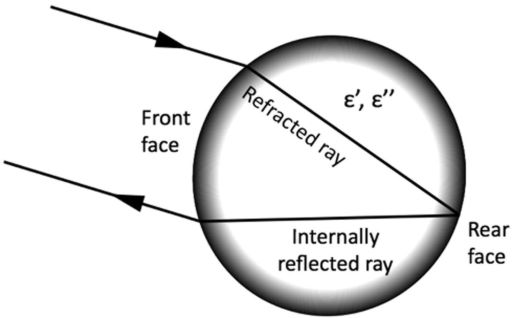
Le Gall et al, Icarus 207 (2010) 948-958
Radar backscattering in a spherical cobble
This diagram explains how a transparent sphere can efficiently scatter incoming radiation back at the radiation source. It doesn't matter what direction the radiation comes from; provided the sphere is reasonably transparent, a significant amount of the radiation will be sent back to its source.Le Gall writes: "Despite the intensity reduction produced at the three dielectric discontinuities," that is, the front of the sphere, the back of the sphere, and the front of the sphere again, "this mechanism can lead to an extreme enhancement of the backscatter when the sphere is transparent enough to preclude significant attenuation on the round-trip between the front and back surfaces. On Earth, water droplets of a particular size produce the optical phenomenon known as 'the glory' which appears like a bright halo surrounding the shadow of an airplane on clouds. For that matter this property of transparent spheres is commonly exploited in the manufacture of reflective paints and tape."
It's a very cool idea. But can it work? I immediately thought of three possible objections. First: the Huygens cobbles aren't spheres; they're round but not perfectly so. Does the geometry still work if the cobbles aren't spheres? Second: does the geometry work for cobbles that are the same size as ones we've actually seen on Titan? And last: are Titan cobbles likely to be radar-transparent enough for this to work?
The next section of the paper addressed all three of these concerns using mathematical modeling. First, Le Gall looked at whether the process works for Titan-sized cobbles. She shows that you would predict very high backscatter for cobbles made of ice or ice plus ammonia for cobbles in the range of sizes observed by Huygens, though it doesn't work well once the cobbles get too big (above about 25 centimeters in diameter). She didn't run through the model for other possible cobble compositions (clathrate hydrates, aggregated tholins, or clumped hydrocarbons), but argued as to why the model should still hold for those other possible compositions.
So that addressed the size and radar-transparent questions I had. Next she explored the problem of non-spherical cobbles, modeling the cobbles as oblate spheroids (squashed spheres). This work showed that you would still expect cobbles to produce the glory effect provided they were not too far from spherical. Even if the cobbles were squashed spheres half as tall as they were wide, you'd still expect to see the glory effect, though it would not be as bright as for spherical cobbles. A real distribution of rocks would likely contain some quasi-spherical ones and some flatter ones.
So she showed that you could get an enhanced radar backscatter from a surface containing ice cobbles of the sizes and shapes seen by Huygens. Wait -- doesn't that mean you'd expect to see enhanced backscatter from the Huygens landing site itself? Here's the one part of the paper that has left me scratching my head: the measured backscatter at the Huygens landing site is pretty low, measured to be -2, -2.5, and -4 dB for look angles of 9.5, 16, and 18 degrees, respectively. Using the density of cobbles observed at the Huygens landing site and running through her model for spheres of pure water ice, Le Gall would actually predict the Huygens landing site to have a backscatter of 3 dB. How does she explain this?
As expected, this is far beyond the measured values. Yet, it is important to note that this value is lower than the highest radar cross-section measured in the T44 channels. This suggests that, if the channels are also areas of collection of sediments, the density of the pebbles in their streambed has to be larger than the density of the pebbles in the Huygens landing site in order to account for the measured high backscatter. Their rock abundance and distribution are difficult to constrain but we expect the sediments to be closely spaced, possibly closely packed and maybe also larger in the channels.
It is certainly true that river beds would be expected to have more closely spaced and larger cobbles than the plains onto which they empty. But it would have been so much neater if the Huygens landing site had had a cooperatively high backscatter. Real geology never works out quite as neatly as our models!
The paper goes on to paint a picture of what the presence of all these cobbles in a river valley next to Xanadu might mean for Titan geology as a whole. There are a few too many story lines in there for me to summarize in this blog entry; I'll just stick with a view of the river itself. Imagine standing on its bank, your feet tracking a fine mud of tarry dark hydrocarbon ooze overlying a stiffer wet sand. Before you stretches out a flat, dry riverbed absolutely covered in rounded cobbles of dirty ice, many of them as big as your head. It's hard to see the far bank, over a kilometer away, lost in the dreary haze. Suddenly, you feel a faint vibration. Hours or even days ago, a once-in-a-millennium storm dumped a rain of methane on some distant reach of northern Xanadu, hundreds of kilometers upstream. Xanadu's valleys gathered the flooding gases into a slow-motion torrent headed in your direction, carrying with it more rolling, tumbling boulders of ice.
You can pogo away from the now-dangerous bank, maybe even rise above it by flapping some manmade wings; Titan's thick atmosphere and Moon-like gravity makes human-powered flight easy here. You'd have more time to escape a Titanian flood than an Earth one because the slight gravity also slows the gravity-driven flow of methane, although this is counterbalanced a bit by methane having a lower viscosity than water.
Eventually, the flooding methane subsides, flowing away or sinking into the ground, and the action is over, leaving behind a new layer of rounded cobbles to create a bright radar glory.
Let’s Go Beyond The Horizon
Every success in space exploration is the result of the community of space enthusiasts, like you, who believe it is important. You can help usher in the next great era of space exploration with your gift today.
Donate Today