Planetary Radio • Sep 27, 2023
2023 NASA Innovative Advance Concepts Symposium: Part 1
On This Episode
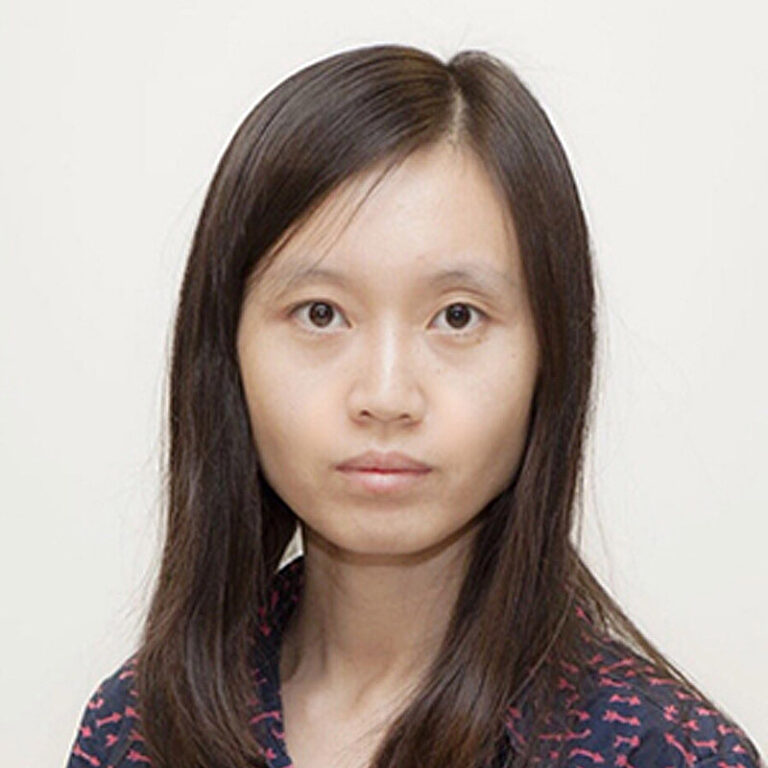
Congrui Grace Jin
Adjunct Professor at University of Nebraska - Lincoln
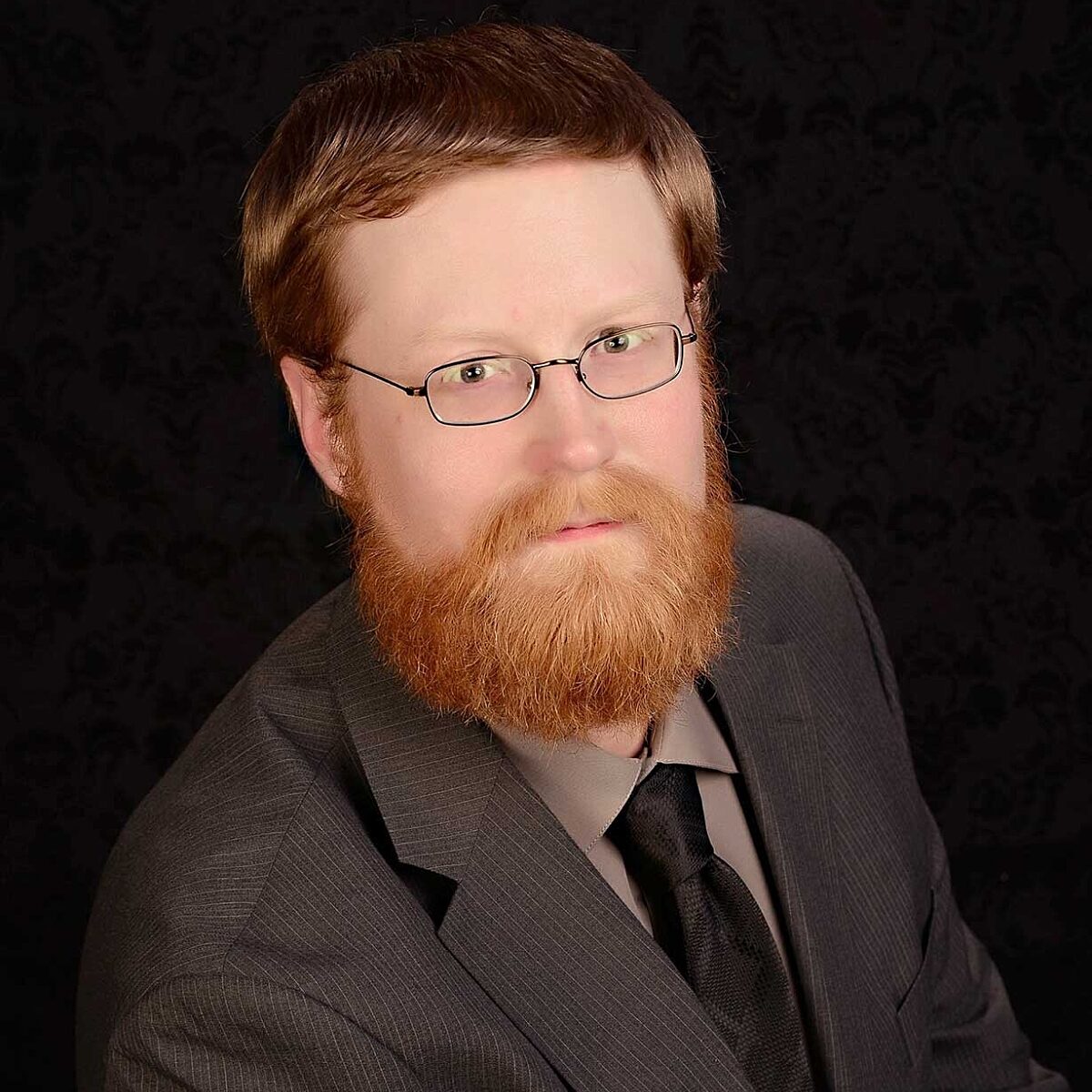
Quinn Morley
Principal Investigator at Planet Enterprises
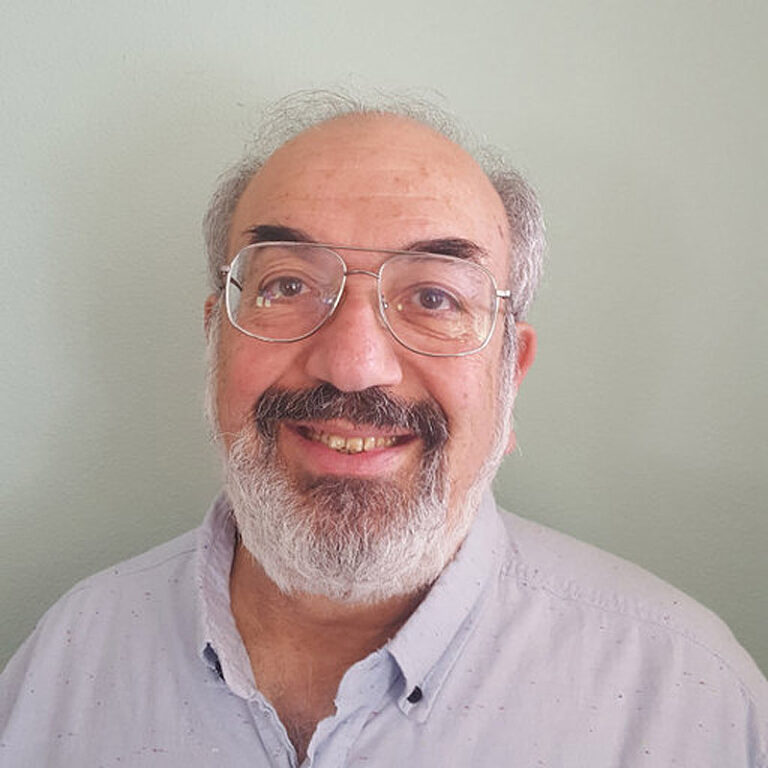
Ronald Polidan
Director of Programs at Lunar Resources, Inc.
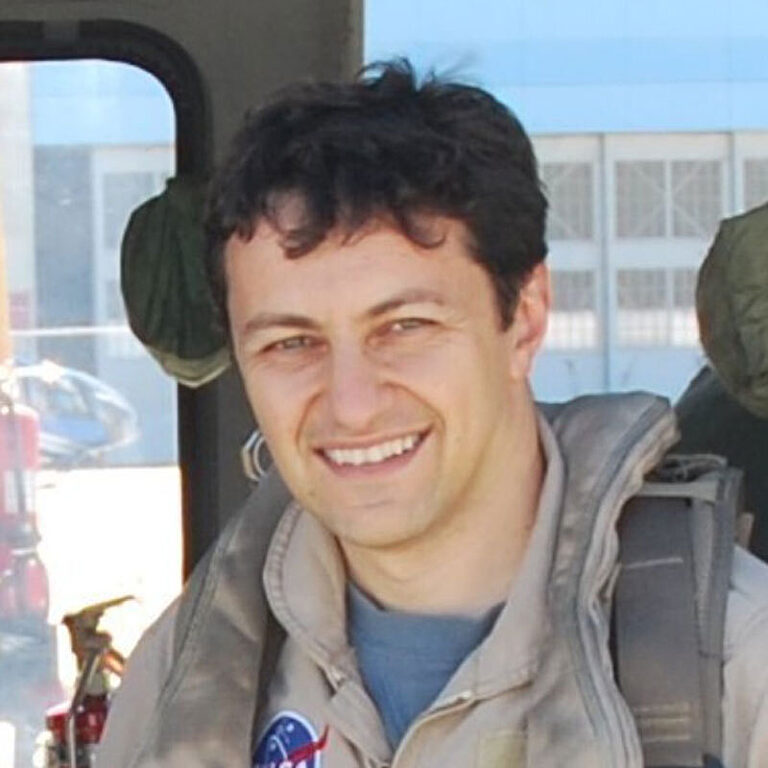
Edward Balaban
Research Scientist at NASA Ames Research Center
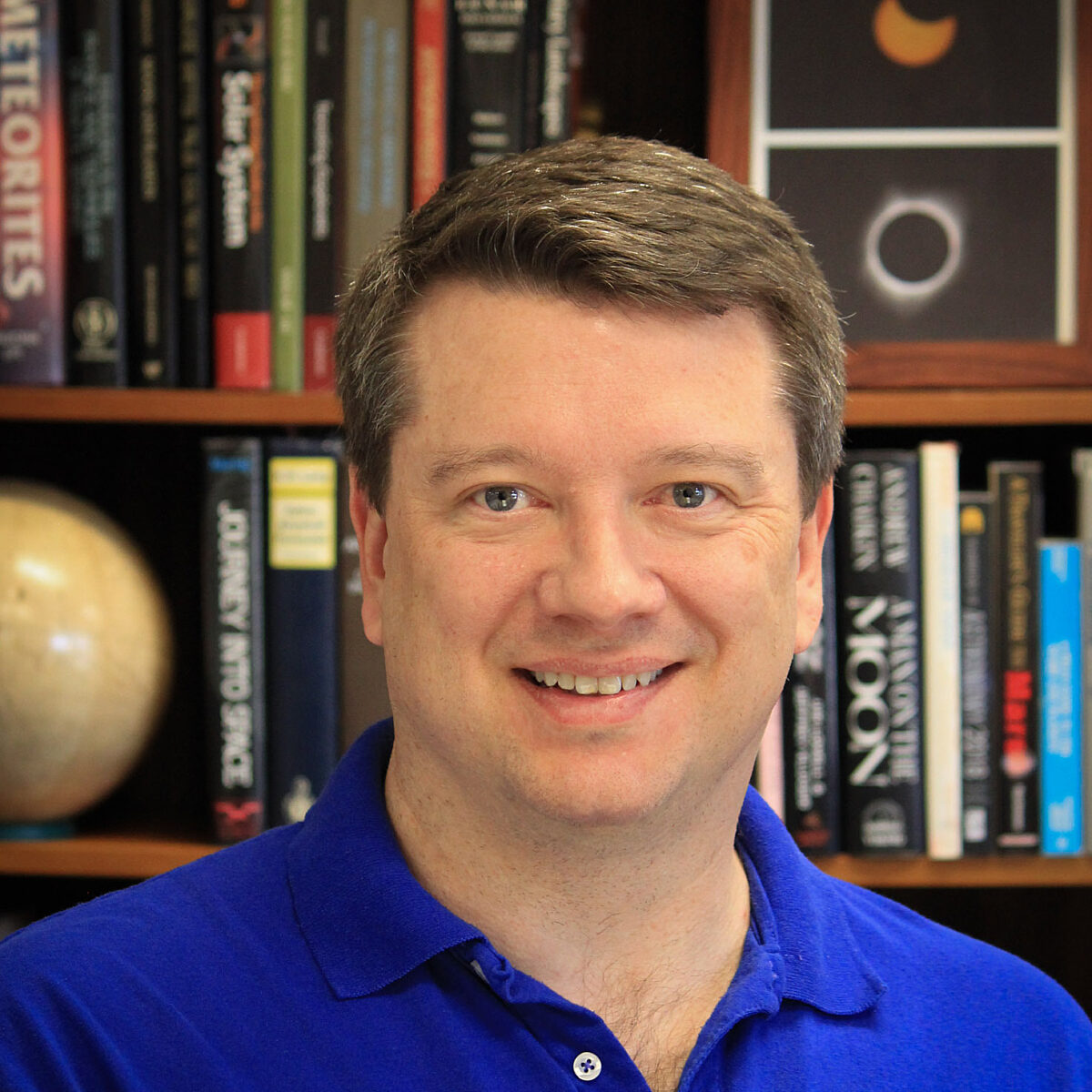
Bruce Betts
Chief Scientist / LightSail Program Manager for The Planetary Society
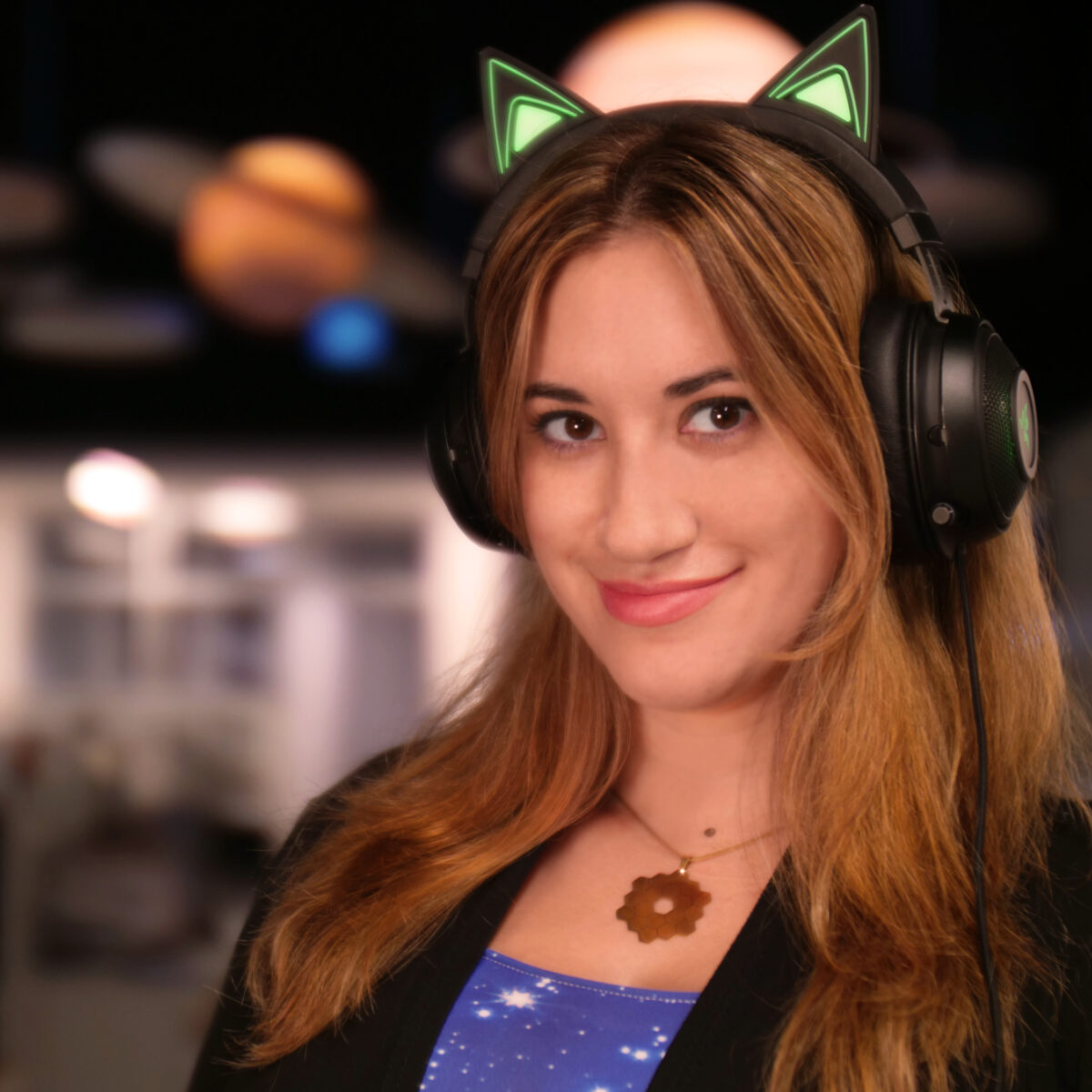
Sarah Al-Ahmed
Planetary Radio Host and Producer for The Planetary Society
Join Planetary Radio host Sarah Al-Ahmed on a trip to the 2023 NASA Innovative Advanced Concepts (NIAC) Symposium in Houston, Texas. In this jam-packed two-part series, you'll hear Sarah's interviews with the inspiring NIAC fellows who are thinking up the technologies that could change the future of space exploration. In this episode, you’ll hear from Congrui Grace Jin (University of Nebraska, Lincoln), Quinn Morley (Planet Enterprises), Ronald Polidan (Lunar Resources, Inc.), and Edward Balaban (NASA Ames Research Center). Stick around for What's Up with Bruce Betts, the chief scientist of The Planetary Society, for a discussion about the advances in space exploration during our lifetimes.





Related Links
- NASA’s Innovative Advanced Concepts Program
- Biomineralization-Enabled Self-Growing Building Blocks for Habitat Outfitting on Mars
- TitanAir: Leading-Edge Liquid Collection to Enable Cutting-Edge Science
- FarView Observatory – A Large, In-Situ Manufactured, Lunar Far Side Radio Array
- Fluidic Telescope (FLUTE): Enabling the Next Generation of Large Space Observatories
- OSIRIS-REx returns sample from asteroid Bennu to Earth
- Make your gift to LightSail’s Legacy
- The Night Sky
- The Downlink
Say Hello
We love to hear from our listeners. You can contact the Planetary Radio crew anytime via email at [email protected].
Transcript
Sarah Al-Ahmed: Dreaming up the technologies of the future, this week on Planetary Radio.
Sarah Al-Ahmed: I'm Sarah Al-Ahmed of The Planetary Society with more of the human adventure across our Solar System and beyond. Join me on a trip to the 2023 NASA Innovative Advanced Concept Symposium or NIAC. This year it was hosted in Houston, Texas. Over the next two episodes, you'll hear my interviews with the fantastic and inspiring NIAC fellows who are thinking of the technologies that could change the future of space exploration. Then we'll check in with Bruce Betts, our chief scientist for What's Up, and a discussion about the advances in space exploration during our lifetimes. If you love Planetary Radio and want to stay informed in the latest space discoveries, make sure you hit that subscribe button on your favorite podcasting platform. By subscribing, you'll never miss an episode filled with new and awe-inspiring ways to know the cosmos and our place within it. Before jumping into NIAC, I have to send a massive congratulations to the NASA OSIRIS-REx mission team. It's been a long three years waiting for the spacecrafts collected samples from the potentially hazardous asteroid called Bennu to reach Earth, but the team did it. OSIRIS-REx's samples touched down on Earth on Sunday, September 24th, 2023. We'll explore the mission and the fate of these samples in an upcoming episode of Planetary Radio. I know you'll all enjoy it. But as of OSIRIS-REx's samples were making their way back to Earth, I was off on my first adventure to the annual NASA Innovative Advanced Concept Symposium this year. It was held from September 19th through the 21st in the Hilton Houston NASA Clear Lake Hotel. That's in Houston, Texas, right on the water by Johnson Space Center. I got to host the webcast for the event, speaking with all of the amazing NIAC fellows as they shared their proposed technologies. The NIAC program is designed to nurture visionary ideas that could transform future NASA missions. They could be new methods of propulsion, intriguing new telescope designs, biotechnologies that could improve the lives of people living and working in space and so much more. The program seeks ideas from diverse and non-traditional sources. That could include everything from teams of university professors and students to intriguing ideas from commercial space companies. The NIAC program awards fellowships in three phases. Phase 1 projects are given nine months to explore the ideas before moving on to Phase 2 and 3, each of which allows the teams two years more to explore their concepts more deeply. The program is highly competitive and not all ideas become a reality, but that's part of what makes this such a fantastic program. It allows NASA to create innovative technologies that would otherwise remain a dream. And I'm telling you, the energy when you get that many creative people together in one space is so inspiring. Let's meet some of the NIAC fellows as they dream big and share their passion for aviation and space exploration. We are here at the 2023 NASA Innovative Advanced Concept Symposium here in Houston, Texas. It's my first time here. We're right down the street from the Space Center Houston. I'm really excited to be here. The main premise of this is that dreams are the beginning of what takes us out there, what allows us to innovate and then go out into space. We have these ideas in our hearts. And the people here today are the ones that take those ideas and build them into reality so that we can then carry those technologies out into space with us. There's some really exciting things coming up in our future. I'm here with Congrui Jin from the University of Nebraska Lincoln. You have some really cool innovative ideas about new ways to build habitats on Mars. Would you like to tell us a little bit about that?
Congrui Grace Jin: Yeah. The idea is to use cyanobacteria and the fungi to make them produce biominerals, which is calcium carbonate, which function as glue to bind the soil particles on Mars together to make building blocks. We can build them just using those microorganisms.
Sarah Al-Ahmed: Why is it so challenging to build on Mars that we need to suggest these alternate technologies for building materials instead of just going there and doing what we would normally do, build a habitat out of things that we bring from Earth?
Congrui Grace Jin: Yeah. If you bring materials from Earth and transport them to Mars, that will be very expensive. So that's why we use microbes because you can just take small quantities of spores to Mars and then they will be reproducing themselves based on the in situ materials already available on Mars like air, sunlight and so on.
Sarah Al-Ahmed: How does this cyanobacteria pair together with these filamental fungi to actually bond together materials? How does that process work?
Congrui Grace Jin: If you find the right species or strains, they are able to support each other. So cyanobacteria, basically they will do photosynthesis. They will convert carbon dioxide and they will fix nitrogen and convert them into organic matter to support and assist the growth of fungi. And fungi will provide protection and the minerals also the CO2 to support the cyanobacteria, so they will rely on each other and grow simultaneously.
Sarah Al-Ahmed: See, that's really interesting because here on Earth there's a lot of interest in carbon capture and these kinds of technologies. We don't necessarily have to worry about that on Mars, but are there applications for this kind of technology here on Earth that can allow us to build habitats that might help us clean out our air a little little bit?
Congrui Grace Jin: Yes, definitely. Actually, my research group started on the project of self-healing concrete. So we use this technology to heal cracks in concrete and we see them producing biominerals, which can heal the cracks in situ automatically. So we want to take this one step further to make self-growing structures to build structures on Mars.
Sarah Al-Ahmed: Self-healing concrete is actually a really important thing here. I know we've been seeing some interesting research into what happened with Roman Concrete, how that was self-healing. And a lot of our carbon emissions come from our concrete buildings, so this is actually a really cool solution, not just for Mars, but here on Earth, so that's really awesome. But Mars has some very interesting chemical situations that are not present here on Earth. For example, the regolith is very high, and perchlorates, all kinds of different chemical situations we don't deal with here. How are you going to be testing this technology to make sure it actually works with Martian materials since we don't have samples?
Congrui Grace Jin: Oh yeah. We use Martian regular stimulants. We assume that the stimulants truly represent the real regulars, but this may not be true. But right now that's what we can do. So we grow cyanobacteria and fungi together on Petri dish with the presence of Martian regular stimulants. We did observe some of the strains can grow very well. They can support each other. But most of them will die because of the high pH value of the components that will kill most of the microorganisms.
Sarah Al-Ahmed: What kind of structures can you actually build with this technology? Because I can't imagine that you'd be able to just build a whole house out of this. You would have to take it in little chunks.
Congrui Grace Jin: Yeah. So we need to make molds and put the mushroom regulars particles into the mold and the cyanobacteria and fungi will produce the glue to bind those particles together into a cohesive body. And then you can take all them from the mold and that will be one building block. And then based on the building block, you can make walls, floors, and furniture like tables and chairs for the people who are working on Mars.
Sarah Al-Ahmed: How do you build the basic structure? Because you're going to need to build that structure for them to grow over. Do you use things like 3D printing structures that you then grow over or are people going to have to be there to actually build these underlying structures first?
Congrui Grace Jin: So using this technology, we can do building blocks, just bind those granular solid particles into a cohesive body with reasonable strengths, but later we may need robotics to kind of pile them up to make some furniture.
Sarah Al-Ahmed: Is the idea here that we're going to be doing this once we actually get to Mars? Or are we going to build habitats ahead of time so that when humans get there, they can then habitat in those places?
Congrui Grace Jin: Yeah, yeah, yeah. You can build them before people are there, yeah.
Sarah Al-Ahmed: Mars doesn't have a global magnetic field that's as strong as we have here on Earth. We're going to have to worry about shielding people there so they can be protected. Is there any thought here behind ways that we can build around these habitats to help shield people or is that a separate technology that we're going to have to think up on the fly later?
Congrui Grace Jin: Yeah, I think we also have this problem. We have to shield those microorganisms because they cannot directly survive on the natural condition. So right now what we plan to do is to have to use a bioreactor which can shield the harmful atmosphere to protect them so that they can produce those biominerals.
Sarah Al-Ahmed: How airtight is this material? Because in order to actually put people in these places, we're going to have to make sure that we can aerate the inner area and keep it separated from the Martian atmosphere.
Congrui Grace Jin: Yeah. They have to have really good strengths because we are using them for construction purpose. They also need to have very good radiation shielding properties. Yeah, those are the challenges we are currently have.
Sarah Al-Ahmed: That's a really cool technology and a really interesting way of thinking about it. I know there are a lot of people who have suggested in the past using fungus and things like that to build habitats on Mars. But adding this extra element of these two things together, the cyanobacteria along with the fungal filaments I think is a cool way to do that. I'm wondering if we can up this technology for places like the moon as well because we're going to have to go to the moon before we can go on to Mars and test all those technologies ahead of time.
Congrui Grace Jin: Definitely. I want to point out that previously people mostly use the fungi filaments, like those polymer type materials to make construction. They are more like lightweight composites. But right now we are using them to produce biominerals, which is more concrete like materials, so they have better strengths and also they are different from the previous technology. What we want to do here is to make brick-like materials like concrete or the materials that we can build houses. So it's a little bit different. Yeah, I think it definitely can also be applied to moon. But the moon and Mars, they have different atmosphere like the CO2 and the nitrogen, so it will be a challenge to be used in our moon. Right now our target is Mars.
Sarah Al-Ahmed: Well, I mean Mars is the most forward-thinking of these things, and that's what NIAC is here to do. We're not just thinking about what we're going to be doing tomorrow, we're thinking about what we're going to be doing decades in the future. So keeping our eyes on Mars is a good way to do this. And then maybe you can do the same experiments with lunar regolith. We already have samples of that, so it might be a little easier.
Congrui Grace Jin: Yes, yes, definitely. Thank you so much.
Sarah Al-Ahmed: We are here with Quinn Morley from Planet Enterprises. I wanted to congratulate you because I understand this is your second NIAC fellowship. Is that true?
Quinn Morley: That is true. I won both of them as an undergrad. And a lot of that was really the inspiration came from Planetary Radio actually. So that's sort of what set my career transition in motion.
Sarah Al-Ahmed: That's really heartwarming to hear. Planetary Radio has been going on for 20 years and our former host Matt Kaplan was an absolute star. But anytime I learn that people are a fan of our podcast, of our show, it really warms my heart, so thanks for saying that.
Quinn Morley: No problem.
Sarah Al-Ahmed: I love your concept. You are proposing a new way of exploring Saturn's moon, Titan. We're already looking forward to the Dragonfly mission, which is going to be more of a rotor craft, but you are proposing a different way of flying through Titan's atmosphere. What's your idea?
Quinn Morley: Okay, so my idea centers around the effect we see when we fly on an airplane on Earth. If you fly through a rainstorm or if you fly through a cloud, you can see water on the wing or going by the window. And the idea is to just drink that in through the airplane skin and then do science in that liquid. So not even actively sampling, just taking it right through the skin through a permeable surface. And where we differ from Dragonfly is we want to target the areas of Titan that are going to have rain clouds, lakes, that kind of stuff. Dragonfly is focusing on the drier regions, so it reduces their mission risk. And so we're really going for the risky stuff to try and do the hard science.
Sarah Al-Ahmed: Is that what inspired this idea, just kind of sitting out an airplane here on Earth and seeing the condensation on the windows? How did that idea begin?
Quinn Morley: Yeah, I think I wanted to do a Titan airplane concept originally. And I also love capillary effects and it's just something I seized in my everyday experience, which I guess is rare apparently. And so I put the two together after thinking about it for a while.
Sarah Al-Ahmed: How large would this Titan Air aircraft be? Because I'm trying to just conceptualize. You don't need a huge vehicle, but you could with the density of the atmosphere on Titan.
Quinn Morley: So it's going to be about the same size as the PBY Catalina. So 18 meters in length, and that's to fit in Starship, and a 25-meter wingspan and about a 3-meter fuselage, and that's sort of four thermal requirements using passive RTGs like the normal RTGS we use on emissions. We use multiples of those so we can up that power output. And that lets us charge batteries while we're floating on the lake and later we can take off and go up and do science, get to the cloud, the rainmaking clouds at 30 kilometers if we need to.
Sarah Al-Ahmed: Yeah, I was going to ask, so it's powered conceptually by radio isotope, thermoelectric generators. Titan is an interesting place in that it has a whole hydrological cycle made out of hydrocarbons. So hypothetically, you could use that as a fuel source, but I think it makes more sense to instead just test those materials and use an RTG or something to power yourself.
Quinn Morley: The problem with using hydrocarbons as power on Titan is there's no oxygen. It's locked up in the crusts. You'd have to harvest it out of the ice in order to use to burn the fuel. Or you could bring oxygen with you, which isn't an option, I guess. I hadn't thought about that. But you could probably bring solid oxygen somehow or an oxygen generation system like we use on airplanes for the gas masks and you could produce it if you needed it that way.
Sarah Al-Ahmed: But this craft would actually land on liquid, not on land. Is that too protected as you go up and down? Because otherwise you're going to need some little feet or some kind of runway or something to land, right?
Quinn Morley: It just seemed like an elegant solution to me. And also if we're doing the hydrological cycle, now we're on the lake, it's convenient because we can get that late stage where it flows into the lake so we can look at the whole cycle. And so that just seemed like a good way to do it. It'll just be a relocatable land or lake Dragonfly also. We can move around from lake to lake or different places in the lake or come back to our favorite one every time. And if we didn't have that ability, a lot of the other flight concepts are perpetual flight really, so we can land and do science and we can expend power on things like climbing. The other concepts really couldn't do because they're trying to stay within their tight energy budget.
Sarah Al-Ahmed: So as this vehicle is flying through the air, it's soaking in this material from the rain, is it going to be able to determine what chemicals are in there, what kind of organic compounds are happening? What kind of science are you excited to do with it?
Quinn Morley: That's actually the real driver, is to figure out what is in the aerosols in Titan that form the clouds. So the clouds on Earth when they form, each particle of the cloud is formed around an aerosol, which is usually Sahara and dust or ocean spray, stuff like that that is the nucleus for the raindrop or the part of the cloud, right? And so we want to see on Titan, maybe that's an amino acid. And so we could just collect the rain and just send that to a continuous science suite, which is sort of inspired by the way we analyze ice cores on Earth. We melt the liquid and continuously analyze it as we melt the ice cores slowly and just analyze the continuous fluid stream.
Sarah Al-Ahmed: I think this is really cool too because there are a lot of people that are really interested to learn more about the potential river deltas and stuff that run into the lake districts on Titan, but we have such limited data on this from the Huygens probe and the Cassini mission. There's a lot that we don't understand. So is this craft going to have a slew of cameras underneath or are you focusing mainly on identifying these aerosols?
Quinn Morley: I think that that's really an easy add-on, is to add cameras and stuff to it, even radar so you can image the coastline really closely. Because that would be an easy flight. You could be a one kilometer up, maybe fly for an hour and then learn something and figure out where you're going to fly next time. I think that we would want to include as many science payloads as we can having a larger aircraft, and just that our technology focuses on that capillary collection, but what else could we have? And then realistically, we would probably be part of someone else's mission, right? Like a technology demonstrator perhaps on a Dragonfly clone that could target the wetter regions. Maybe if it had pontoons or something, it could land in an area that's muddy or wet and we could have a small section of the skin dedicated to testing our system.
Sarah Al-Ahmed: This is a really cool concept because I've always wanted them to target specifically these lakes and these rivers, but that's a dangerous thing for most spacecraft. So this is a really cool concept to actually get that done. And I'm glad that you're thinking about it because of all the worlds in our Solar System, another world with a hydrological cycle with lakes and rivers, it's such a beautiful target and could really expand our ideas of what's going on with exoplanets.
Quinn Morley: It's really incredible to think about Titan. And there's other crazy things we've learned about Titan. Just the other day I was doing the math for how we would have to get up on plane on the lake before we could take off like if you see a flying boat on Earth. And it turns out that we'll be at our cruising speed for flight before we even hit that planning speed. The planning speed's twice where the cruising speed and flight is, so we're just going to get lifted up out of the lake by the wings, so it's just nuts.
Sarah Al-Ahmed: That's really cool. What would be the lifetime of this kind of mission? You could potentially go for quite a long time with an RTG as we've seen with the Voyager missions. How long do you think these would work?
Quinn Morley: I think I'm inspired by what curiosity has done with RTG. So if we had perhaps three RTGS that what we're looking at. But the limiting case really is probably structural stuff because it's so hard at the cryogenic temperatures. And we're looking at inflatable wings to fit in the launch vehicle. And so polymer science at 90 Kelvin is not easy. And so the wings realistically would be fatiguing from issues like wrinkles or buckling slowly over time. And so that's probably our limiting case really, is some hardware gremlin from the low temperatures that we're just not able to fully prove out. So I still think one, five, 10 years is probably a year would be like the nominal target at Earth year.
Sarah Al-Ahmed: Well, awesome. Thanks for joining me Quinn. And seriously, good luck with this because I would love to see this work. We'll be right back with the rest of my adventures at the 2023 NASA Innovative Advanced Concept Symposium after this short break.
Bill Nye: Greetings. Bill Nye here, CEO of The Planetary Society. Thanks to you, our LightSail program is our greatest chaired accomplishment. Our LightSail 2 spacecraft was in space for more than three years from June 2019 to November 2022 and successfully used sunlight to change its orbit around Earth. Because of your support, our members demonstrated that highly maneuverable solar sailing is possible. Now it's time for the next chapter in the LightSail's continuing mission. We need to educate the world about the possibilities of solar Sailing by sharing the remarkable story of LightSail with scientists, engineers, and space enthusiasts around the world. We're going to publish a commemorative book for your mission. It will be filled with all the best images captured by LightSail from space, as well as chapters describing the development of the mission, stories from the launch, and its technical results to help ensure that this key technology is adopted by future missions. Along with the book, we will be doing one of the most important tasks of any project. We'll be disseminating our findings in scientific journals at conferences and other events, and we'll build a master archive of all the mission data. So every bit of information we've collected will be available to engineers, scientists, and future missions anywhere. In short, there's still a lot to do with LightSail, and that's where you come in. As a member of the LightSail mission team, we need your support to secure LightSails legacy with all of these projects. Visit planetary.org/legacy to make your gift today. LightSail is your story, your success, your legacy, and it's making a valuable contribution to the future of solar sailing and space exploration. Your donation will help us continue to share the successful story of LightSail. Thank you.
Sarah Al-Ahmed: I have with me Ronald Polidan from Lunar Resources Incorporated. Thanks for joining me.
Ronald Polidan: Oh, thank you for inviting me.
Sarah Al-Ahmed: And congratulations on making it to phase 2. That's got to be really exciting.
Ronald Polidan: It is. Very exciting. We're very pleased with this.
Sarah Al-Ahmed: So your project is a proposal for studying the cosmic dark ages with a really cool telescope on the far side of the moon. Before we get into the details though, can you explain a little bit about the cosmic dark ages and what that is for the people online?
Ronald Polidan: Sure. When the universe formed after the Big Bang, all that was around was hydrogen and helium. It hadn't formed stars yet. So it takes some time for those to coalesce into the first stars. So for that first period, the universe was just full of hydrogen and it was dark because there were no stars to illuminate it. And then as the stars started forming, the universe eventually became transparent and the universe we see today. So what formed in that first epic of dark ages was all the structures that led to all the galaxies. So we don't know how that happened. We see stuff from web today that is saying it's a lot different than we were thinking. And so, the goal of this observatory is to really understand how those first structures formed and how the first galaxies and stars came into existence.
Sarah Al-Ahmed: So the FarView Observatory is a concept of putting a radio telescope array on the far side of the moon. Why is this specific range of wavelengths of light something that could allow us to see back to this early part of the universe?
Ronald Polidan: It's the redshift, the hydrogen atom emits at 21 centimeters, and that's in the nearby environment. But if we go back 13.8 billion years, it's been redshift. And it gets redshift into the very low radio frequency, so 50 to 5 megahertz. And so we're still looking at normal hydrogen except it's been so redshift that it's now into the very long wavelength radio.
Sarah Al-Ahmed: It would be really wonderful to know more about this period in time in the universe because as we're looking back into the universe with things like the James Webb Space Telescope, there are just parts of this timeline and space that we really don't know a lot about. And I'm not sure how many people are familiar that there's this whole time period before the stars finally started shining their light through the universe. It's a really interesting time period, but in order to do that, we need a telescope. So why do you want to put this telescope array on the far side of the moon? How does that allow us to do things we wouldn't be able to do otherwise?
Ronald Polidan: Well, there's two reasons. One is that we cannot actually cannot observe those wavelengths from the Earth. The ionosphere cuts them off, and so they never penetrate the Earth's atmosphere. So ground-based telescopes can't see them. But also the far side of the moon, the Earth itself generates a tremendous amount of radio noise. And the far side of the moon, because the moon is nicely solid and opaque, it blocks all that noise. And so it actually is the radio quietest part in the inner Solar System. So we will have very low backgrounds. And this signal from the early universe is very, very faint. And so we need that in order to be able to detect it. But also we detect other things, all the planets in these bands, Solar, coronal mass ejection permit. So this is a very broad-based telescope, but all of it needs to get away from the noise that the Earth makes.
Sarah Al-Ahmed: You're proposing building this with in situ resources, building it out of stuff that we actually find on the far side of the moon. How are you going to accomplish that and what kind of materials are you looking for in order to do this?
Ronald Polidan: Well Lunar Resources, the company I work for, has developed technologies that can take lunar dirt, lunar regolith, and break it apart. The lunar regolith is mostly metal oxides. And so we break those apart into oxygen and metals. And so we generate a lot of aluminum, magnesium and other metals. We purify them and we end up with basically pure aluminum is our primary product. We build the antennas, the power lines out of those aluminum. We also get silicon out of this and we generate Solar cells. So we actually are self-sufficient in that once we get going, we start building our own power system and then we start building our arrays and then we can build batteries to power them at night. And so the whole thing is living off the land in the classic sense.
Sarah Al-Ahmed: How are you actually going to gather these materials? And where are you looking to put this that has the abundance of materials that you need?
Ronald Polidan: Well, we have actually a group of colleagues in India that are actually using data from their satellites to identify spots on the far side with very high aluminum content, because we need the aluminum content to be as high as possible in order for us to build this in a reasonable amount of time. So once we find those sites, then I go in and look at them to see we have a bunch of parameters that are needed. They need to be flat, they need to be within this radio quiet zone. So there's a number of factors that come in. And then we basically dump the dirt into our low reactors and they get processed by electrolysis. One spigot comes a bunch of oxygen, which we don't need, but everybody else wants. And then the metals come out of another area and we refine those and we're ready to start putting aluminum pellets or aluminum wires or whatever we need. And then a rover picks those up and we have another technology that can vaporize that. We deposit our antennas and our wires directly on the lunar surface. So they're basically just laying on the dirt.
Sarah Al-Ahmed: So we've got rovers collecting these materials, putting them into these things to generate the materials that we need. How do all of those things, all the wires, finally become this telescope array? Are we going to need humans to do it or can we still do it with robots?
Ronald Polidan: It can be done with robots. And that's the major part of the study, is all the pieces are fine. We understand all the little pieces. But trying to fit all the pieces into a system that actually closes is non-trivial. All of these features impact each other. So if I have a rover that's a little slower, then it's going to slow everything down. If my extraction rate of aluminum, it goes down, then that's going to impact things. So it's a major system of systems. And so trying to fit all that together is really the key. And so it's looking at what constraints do we put on things even from the standpoint of how we position the dipole antennas. The antennas themselves are basically, if you had one your hand, it looked like a strip of aluminum foil, so it's not exactly impressive. We have another technology that may work better and it's just going to be a wire. So then putting those things down in some pattern that is consistent with what science needs is going to be the real issue.
Sarah Al-Ahmed: And once you get this whole thing constructed, how do we then get the information back from these telescopes? They're on the far side of the moon, so they're going to need to communicate with us somehow.
Ronald Polidan: Right. And we generate a lot of data. There's a hundred thousand dipole antennas, and so they generate a tremendous amount of data. And that's one of the big issues for our phase 2 study, is how to get that quantity of data down to something manageable. But manageable means even then we will need to have optical communication link. So there will be something in lunar orbit which will communicate with our antenna on the ground, and we'll send up the data optically and then they'll relay that back to the Earth. But the data management problem is fairly severe.
Sarah Al-Ahmed: Yeah, it's not like we have wifi on the far side of the moon. We're going to need a large number of satellites, especially as we start taking humans to the moon and perhaps someday to Mars. We're going to need a much more robust communication system, especially with telescopes that are now beaming down an incredible amount of data. That's a real challenge.
Ronald Polidan: Correct. We are actually taxing the limits of analysis because this is much bigger than any ground-based telescope. We cover 225 square kilometers, so it's about the size of the city of Houston. That's a lot of antennas to maintain. But the one advantage we have is because we're in situ built, then if something fails or some problem develops, we just go repair it. So we have a possibility of having a 50 year, 60 year old lifetime for this because we'll just go repair things as they break. And that's the big advantage of in situ. Once we're up there, we don't need anything else from the Earth.
Sarah Al-Ahmed: You are exploring all these materials in situ that we can use, but are you also developing the rovers that are going to go along and build this thing as well?
Ronald Polidan: No, we're actually using other companies to develop those, right? Our phase 2 is teamed with Lockheed Martin. We're going to baseline one of their rovers as our sort of test particle rover. The goal of that study is, what do we need the rover to do? And this is a fairly advanced concept rover, can it actually achieve that? So it'll give us a much firmer grip on feasibility for the requirements for the rover.
Sarah Al-Ahmed: Yeah, that's a real challenge, but again, another opportunity for different organizations to collaborate with each other. It's really wonderful seeing this new commercial push because now there are so many more organizations that have the resources to team up in order to accomplish this. Otherwise, we wouldn't have been able to do this in the past. This is a really cool idea.
Ronald Polidan: Oh, thank you. No, we're very excited about it. We think it's got a lot of promise. It also will do one other thing by understanding how we would do large scale institute resource utilization. It's applicable to band emissions, to other science missions because we'll do the pathfinding for what do you need to do to actually have a functional ISRU system on the moon. And that's going to be very exciting.
Sarah Al-Ahmed: Even just the basic premise of being able to mine oxygen as the lunar regolith could make a huge difference. In combination with all the water deposits that we're finding at the lunar South Pole and that kind of thing, we actually have a solid chance of having the resources that we need to make a sustainable human settlement. So this is cool not just for studying the early universe, but potentially for human habitability.
Ronald Polidan: Yeah. And the one thing with FarView is it's going to be the first self-sustainable observatory because all the stuff will be there needed to repair it, continue it. We could also expand it. If after the first round of science comes in, we decide it needs to be bigger, then we'll just go build some more antennas. And so it's a very different approach to a science mission than what has been done where we have to build everything on the Earth, keep your fingers crossed that it works in space. If after the first six months we find out that our antennas need to be longer or something, then we'll just do that.
Sarah Al-Ahmed: How many rovers would it take to accomplish this? Is your vision like one starting off or a fleet of them?
Ronald Polidan: Initially, one. But for optimal efficiency, it'll be somewhere between two and five. There's a trade of how fast do you want this built versus how much is it going to cost to get one of these rovers to the lunar surface. So we can get by with one, it'll be much slower. Two is really kind of nice. Three is optimal. And more we'll just do things faster.
Sarah Al-Ahmed: And to study this point in the universe, how many telescopes do you actually need in the array? How many do you envision starting out with?
Ronald Polidan: Well, the science simulations that'd been done so far are that we need at least 50,000 to do the dark ages. So that's a lot of stuff. And a hundred thousand is sort of... We probably don't know everything about the environment on the moon, so we probably want to have more than less. And so that's why we baseline a hundred thousand. But for other science, like for Solar physics to study the coronal mass injections, we only need a few hundred. So it's one of these things where we will do whatever science is possible when we have the number of arrays. But yeah, a hundred thousand arrays, a hundred thousand dipoles is really what we need.
Sarah Al-Ahmed: That's a really cool project. I am looking forward to a future where we can actually see this thing become a reality. Right now I'm here with Edward Ballaban from NASA Ames Research Center. I saw your presentation yesterday. That was really cool. I'm really happy to meet you.
Edward Balaban: Yeah. Really nice to meet you, Sarah, as well.
Sarah Al-Ahmed: One of the challenges that's really facing astronomy right now is the issue with the size of our telescopes, as we saw with the James Webb Space Telescope and its launch, trying to fit a large telescope into a rocket is very complicated. You end up folding it up and having a lot of complexity to its deployment, but you're suggesting a new way to get big telescopes into space, a fluidic telescope called FLUTE. Can you tell us a little bit about how this concept works and what it might allow us to do?
Edward Balaban: Absolutely. The problem that you highlighted is exactly the problem that we're trying to solve. Our concept is based on using liquids to form the optical surface and space. And because liquids can be brought into space in a tank, they don't need a particular launch vehicle geometry. We can even bring the liquids in parts, so in several launches if we want to build a really large telescope. And what liquids also give us is using our approach, we can get a continuous optical surface rather than a segmented mirror like James Webb has. Another key point about our project is that the physics that we're using are really scale in variant. So the same physics apply, whether it's a small component, 10 centimeters or 10 kilometers in diameter. So it becomes more of an engineering challenge to build larger and larger mirrors rather than a real technological barrier.
Sarah Al-Ahmed: Yeah, we've definitely seen the success of JWST with the segmented mirror, but there's a lot of complexity there to getting all those pieces working together. What is the benefit of having one giant unsegmented mirror versus this segmented approach that we've had to take?
Edward Balaban: Right. Well, you get optical effects from if the segments are not perfectly aligned. So you get a better quality image with a continuous solid surface. Another thing that we may potentially do in the future but we're not addressing in the current concept is that with a mirror that remains liquid, we may be able to dynamically change its surface and change its focal length or even do more elaborate shape modifications.
Sarah Al-Ahmed: That's a really cool idea. A telescope you can change the shape of on the fly. What kind of fluids would you have to put onto the surface of this telescope in order to get the reflectivity that you need?
Edward Balaban: So we started out with gallium alloys as our primary liquid candidate. We investigated them for a while. They had a lot of attractive qualities for us. Gallium and gallium alloys are highly reflective, even more reflective than mercury in fact. They are non-toxic and they can remain liquid at low temperatures. So you can hold pure gallium in your hand and you can actually go out on the web and buy it and it will melt. There are some alloys that remain liquid up to -18 degrees Celsius. But after some experimentation, we saw that they're a little bit difficult to work with. First of all, they oxidize very easily, not a problem in space, but preparing them for lunch would little bit challenging. Another issue is that they're corrosive, right? So when they come into contact with aluminum, for example, they just kind of bond with it, eat through it. So not the best quality. They're also heavy. So we would need a lot of launch power to lift enough gallium alloys to form a mirror. So lately we've been focusing more on an alternative class of liquids called ionic liquids. And if you've never heard of them, they are really amazing compounds. They're essentially molten salts. They exist in a wide variety of configurations. Most of them have properties that are very attractive to us. They can remain liquid at extremely low temperatures. They have negligible vacuum vapor pressure, so they don't evaporate in space. They're chemically stable in space. The only downside is that they're not very reflective naturally, and therefore we're working on ways of enhancing their reflectivity, developing methods how to create surficial layers, reflective layers, using reflective nanoparticles like gold or silver nanoparticles.
Sarah Al-Ahmed: That's a really clever way to get around that. I know yesterday someone asked you about the telescope and whether or not you were considering using something that would then freeze onto the telescope. Could you talk a little bit about why you've decided to remain with fluid on it instead of actually allowing this to freeze onto your telescope over time?
Edward Balaban: We originally actually started with the idea of forming the mirror and then solidifying it so that we get a stable shape. We don't have to worry about vibrations or we can slew the telescope at any speed we want. But solidifying materials at such large scales is challenging to do it uniformly. We did some experiments with gallium. Especially when doing it inside a frame that constraints the liquid into a certain shape, gallium tended to expand when solidified and we would get these spikes of gallium on the surface, which is obviously not desirable. So we still may, if we find a material that has negligible shrinkage or expansion ratio when solidified, then solidification may become a viable approach. As I mentioned, it has some attractive qualities. On the other hand, by retaining the mirror in its liquid form, we also get some benefits such as that dynamic shape changing that I just described. And also there'll be more tolerant to damage by micrometeorites, for example, which James Webb's mirror already experienced several occurrences.
Sarah Al-Ahmed: Thankfully, all of the different mirror segments on JWST have these little actuators behind them that allowed them to reshape the telescope, but this is an actual thing we do have to worry about. And I'm glad that you actually did these experiments and figured out that it would get all spiky because diffraction spikes we can deal with, not so much spiky gallium mirrors. Yeah. I am wondering though, how thick of a film of fluid do you get on this thing in order to get the reflectivity that you need? Because I imagine if you need a thick film, you would need a lot more fluid and that might increase the weight of this spacecraft.
Edward Balaban: Right. We're thinking just a few microns of the reflective layer. And in our current concept, the reflective layer is actually pretty thin, especially compared to the diameter of the mirror. We're thinking somewhere between 5 millimeters and maybe 20 millimeters of the layer. There are a lot of interdependent parts. We need a thick enough layer to compensate for any local imperfections of the frame. And if we make the frame too perfect, it might become too complex and too expensive to construct. So we need to strike this fine balance between the quality of the frame that we're going for and the amount of liquid that we need to form a layer thick enough to compensate for any local imperfections.
Sarah Al-Ahmed: That brings up a great point, which is that the actual smoothness of the mirror makes a big impact on the quality of your data. Might this allow us to have a smoother mirror than even we could machine because you're working with fluid?
Edward Balaban: Actually, yes. In fact, in our lab experiments when we first solidified some of the polymer based components and then put them under a digital holography microscope, we're pleasantly surprised to see that they had about a 5 nanometer surface roughness. That was a great result because even highest quality professional optics are at about that level, and that's without any post-processing, without trying hard. But then we actually found a pretty easy way of improving that even further, and that's by taking the same component and putting it under an atomic force microscope. And it turned out that we were actually at the measuring limit of the digital holography microscope and the actual surface roughness was below 1 nanometer, which is about the state of the art that you can do with traditional optics. We expect that with a liquid layer in space where we don't have any of the interfacial phenomena that happened during the solidification, we'll basically get a molecularly smooth surface.
Sarah Al-Ahmed: That's really exciting to hear because I know they had some serious challenges trying to gauge how rough the actual mirrors on JWST were when you actually put it in the temperature conditions that would be in space. That's really complex. It occurs to me that there might be people out there that might be wondering to themselves how does the fluid actually stay on the telescope? Those of us that have watched people play around on the ISS with fluids have seen this in the videos, but can you explain how the fluid actually sticks on this telescope?
Edward Balaban: Right. So one condition that we need is that the liquid has a fine properties to the material of the frame. We actually don't need it to stick to the floor of the frame. It just needs to stick to the sides of the frame. We could form a simple mirror just by using a simple ring frame and then putting enough liquid in there to wet the inner sides of that ring and then form two biconcave surfaces. That would be the simplest mirror, but it would be a little bit heavy because we're using probably more liquid than we actually need. So the floor serves as a way for us as another boundary condition to put the least amount of liquid that we need to form the mirror. The spherical shape is the natural low energy state that the liquid wants to take in space. You can imagine a soap bubble if it could remain in space. And if we put a ring on a section of that soap bubble and then remove the rest of it, it will remain in that curved spherical section shape on that ring. And that's the effect that we're taking advantage of here, natural physics of surface tension and capillary effects to get the liquid up the sides of the frame to stay in the right geometry.
Sarah Al-Ahmed: That's really clever. As it's going to be out there in space, you've already said that JWST has been hit by some micrometeorites. It's conceivable that maybe a micrometeor comes through and perhaps perforates that bubble or maybe blasts some of this fluid off into space. Would you need backup fluid in order to deal with that or to compensate if you lose any?
Edward Balaban: We expect that any amount of liquid actually detaching from the mirror as a result of a micrometeorite impact will be negligible. But we can still have a reserve tank of liquid to just inject to restore the original shape if we need to. There might be some gradual evaporation effects over time that we can compensate for and extraneous events like micrometeorite impacts that we can also deal with. But we expect that surface of the mirror will settle relatively quickly after a small micrometeorite hit.
Sarah Al-Ahmed: That's good to hear. There are so many things that telescopes in space have to deal with, these micrometeorites also just cosmic rays and other kind of contamination of the chemicals over time by bombardment from high energy rays. So is that an issue for the fluids that you're looking at, or are they just chemically stable enough that we don't have to worry about that?
Edward Balaban: The answer is that we don't know yet. We're still looking for just the right liquid for our purposes. We're doing a very wide-ranging literature survey at the moment. We may have to engineer our own ionic liquids. And then we'll do extensive testing to see what their stability is to radiation effects and to low temperatures over a long period of time. In fact, as we speak, some of our team members at Goddard Space Flight Center are doing outgassing experiments in the vacuum chamber with some of our candidate ionic liquids.
Sarah Al-Ahmed: That's a good idea. So imagine we actually get these telescopes out into space. What kind of science could this enable that we can't do with our current size of telescopes?
Edward Balaban: Right. We can do high resolution spectroscopy of exoplanets, for example, their atmospheres, which would allow us to answer questions whether they're habitable, and also whether or not there is some life on them, like somebody was talking yesterday by detecting ozone. If we build mirrors large enough, and I think one of our team members computed that at some point, we can even start resolving surface features of those exoplanets around nearest stars. I think he calculated that to resolve features large enough, continent size features at about four light years away, the nearest exoplanets, we would need a telescope with an aperture of about 1.5 kilometers. So yeah, we still got some time to get there, but that gives you an idea of what kind of things we will be able to do with larger and larger apertures.
Sarah Al-Ahmed: And this solves a lot of problems for us. We can't really do that kind of imaging with ground-based astronomy. Some people have suggested some more complicated systems, say using Solar gravitational lensing and that kind of thing to really magnify planets. But this seems like an easier thing that we can get to in a timeline that makes more sense. Because we really want to examine these worlds. I mean, I'm just personally curious about whether or not there's life in the universe, and JWST is giving us a good start. But if we could get the answer to this with something like a fluided telescope, that would revolutionize the way we think of life in the universe. That's really cool.
Edward Balaban: Thank you. Yeah, and that's exactly what's motivating our work, to be able to look further into the universe, look further in the past of the universe and get more information about exoplanets that are now being discovered in dozens every month. It's really exciting. We're discovering a lot of Earth-like exoplanets now. And it would be, personally to me, just incredibly rewarding and interesting to learn more about them.
Sarah Al-Ahmed: Yeah, I did my first undergraduate research on exoplanet finding. The first exoplanets being detected was actually what triggered my entire career into astrophysics. And now we're in an age where we've already discovered over 5,500 exoplanets and we're really just getting started. So we need to be able to categorize these worlds to really understand the breadth of what we're discovering out there. We can't launch a JWST every single week. We're going to need something more low cost. So what kind of scale of finances would we need to build something like this versus something like JWST?
Edward Balaban: We believe according to our current estimates, and we did it as part of our architecture study, our current concept of a 50-meter telescope can probably be done at an order of magnitude lower cost than JWST. It depends on how you launch it, which launch vehicle you use. But we think we can be an order of magnitude cheaper.
Sarah Al-Ahmed: That's really exciting because as much as we all want a JWST out there in space every two seconds, a whole flight of them working together, that's not necessarily feasible. Something like this could be a really great stop gap until we have a whole new set of great observatories out there. So that's really exciting to hear. And I really hope that this project continues and you find the fluid that you need to actually make this work because this could change a lot for us, I think.
Edward Balaban: Finding the right fluid will be one of our key objectives going forward. And we're investing a lot of time into doing this extensive literature search. We're also investing time and resources into starting a computational method of using machine learning algorithms and artificial intelligence algorithms on both designing reflectivity enhancement methods and also potentially designing ironic liquids with the right properties for us. It's saves us time that we can devote to more creative tasks that machines are not yet as good at.
Sarah Al-Ahmed: And hopefully to analyzing all those worlds that conceivably we'll find with these new fluidic telescopes. I have so many more creative NIAC projects left to share. You'll hear more of their innovative ideas in our next episode of Planetary Radio. In the meantime, you can learn more about the symposium and the NIAC program at nasa.gov/niac. Now let's check in with Bruce Betts, the chief scientist of The Planetary Society for What's Up. Hey, Bruce.
Bruce Betts: Hey, Sarah.
Sarah Al-Ahmed: So we're recording this early because as everyone just heard, I'm at NIAC in Houston this week. Lots of cool new bits of space tech, things to think about in the future that could either completely change the way we do space exploration or...
Bruce Betts: Not work at all.
Sarah Al-Ahmed: You know, just be a cool thing that we think about and potentially write in sci-fi books.
Bruce Betts: There you go.
Sarah Al-Ahmed: Is there any one specific bit of space tech that seemed like a dream when you were younger or when you were first studying stuff in college but is now revolutionizing space exploration?
Bruce Betts: Oh, you surprised me with this one. As much as anything, just the advancements in computers and technology and miniaturization is the first broad thing that comes to mind that just in general was a little science fiction seeming a few decades ago. And so now that you can do so much, everything you can do in almost any field is so much more powerful. How is that? Does that sound slightly coherent?
Sarah Al-Ahmed: Yeah. I did want to share this question with you. David Finfrock from Texas USA wanted to ask, "Does Bruce ever get tired of trying to come up with new ways to say random space facts?"
Bruce Betts: Yes. Well, I don't get tired. I get challenged and then I feel badly when I'm not creative. And as we've noticed when we switched to this new format, I just keep forgetting to do it, which is horrifying to me. But yes, definitely, it's hard. So if people want to write into Sarah with ideas for someone who's vocally challenged, then I will do so. What should we do today, Sarah? You could give us the random space fact chant.
Sarah Al-Ahmed: Oh no, but see, I'm emotionally unprepared for that.
Bruce Betts: Oh yeah. Well, I'm unprepared for most of the questions you ask me. But here we go. I'll take it if... Are you good? You can go for it.
Sarah Al-Ahmed: Yeah, you can take it.
Bruce Betts: Okay. Random Space Fact.
Sarah Al-Ahmed: See, I could never match that, Bruce. But all right, what is actually our random space fact this week?
Bruce Betts: Right. So did you ever wonder, or maybe you just know Sarah, what the first moon discovered by a spacecraft was? I mean, by using data from a spacecraft as opposed to from Earth or using telescopes what the first moon was discovered, thanks to a spacecraft.
Sarah Al-Ahmed: I have no idea.
Bruce Betts: It was Adrastea. I may not be pronouncing it right as usual. Jupiter, one of the four moons that are inside the orbits of the Gallant satellites and that are the sources largely for Jupiter's rings, from dust getting blasted off these tiny moons. Three of them were discovered in the time of Voyager 1 passing through. And I believe Adrastea was the first to be announced, wasn't necessarily the first images that appeared in, but then Phoebe and Metis, those all small inner moons of Jupiter. And that started a whole new wave of spacecraft discoveries. And now we're doing telescopic discoveries like crazy. And I noticed the JWST image of Jupiter, they actually imaged Adrastea, which it's only like eight kilometers by something. It's tiny, but they got it along with the ring and other good stuff.
Sarah Al-Ahmed: It's like I know how JWST does it, but I just don't know how it does it. It just keeps blowing my mind. I literally went to go see that thing in person before it launched. I saw it with my own eyes. It was ginormous. It's not like it's surprising it can do this, but it's still shakes me to my core what it's capable of.
Bruce Betts: You're in the right field.
Sarah Al-Ahmed: Yeah.
Bruce Betts: Yeah. No, it is an amazing technological marvel. I use the less scientifically approved term of crazed. It is way crazed in terms of the design of that puppy. And it works beautifully. And that was the real question, I think, in a lot of people's minds. But it's awesome.
Sarah Al-Ahmed: That's the cool thing. It's one of those cases where the dream was almost nearly impossible. When you think back on it, there were so many points of failure and then it totally worked and crushed it. So who knows? Who's to say that some of these NIAC technologies won't actually work?
Bruce Betts: Oh, me.
Sarah Al-Ahmed: We always need a skeptic, Bruce.
Bruce Betts: Only some of them. Some of them have a definite chance. No, I hope they all work. I mean, that would be awesome. But yes, I'm a skeptic. Or at least I prefer the term realist.
Sarah Al-Ahmed: You know, the classic. Extraordinary claims require extraordinary evidence and extraordinary technologies require extraordinary engineers and an extraordinary amount of luck.
Bruce Betts: And a lot of testing. A lot of testing. They don't require that, but it sure makes it more likely that it's going to work.
Sarah Al-Ahmed: As long as there's cake at the end of the tests.
Bruce Betts: Wow. Okay.
Sarah Al-Ahmed: Sorry, Portal reference.
Bruce Betts: All right, everybody. Go out there. Look up the night sky and think about Sarah playing Portal 2. Thank you. Goodnight.
Sarah Al-Ahmed: We've reached the end of this week's episode of Planetary Radio, but we'll be back next week to share more interviews with the NIAC fellows. You can help others discover the passion, beauty and joy of space science and exploration by leaving a review or a rating on platforms like Apple or Google Podcasts. Your feedback not only brightens our day, but helps other curious minds find their place and space through Planetary Radio. You can also send us your space thoughts, questions, and poetry at our email at [email protected]. Or if you're a Planetary Society member, leave a comment in the Planetary Radio space in our member community app. Planetary Radio is produced by The Planetary Society in Pasadena, California, and is made possible by our members with big dreams for the future of space exploration. You can join us and help inspire the technologies of the future at planetary.org/join. Mark Hilverda and Rae Paoletta are our associate producers. Andrew Lucas is our audio editor. Josh Doyle composed our theme, which is arranged and performed by Pieter Schlosser. And until next week, ad astra.