Color-Shifting Stars: The Radial-Velocity Method
The radial-velocity method for detecting exoplanets relies on the fact that a star does not remain completely stationary when it is orbited by a planet. The star moves, ever so slightly, in a small circle or ellipse, responding to the gravitational tug of its smaller companion. When viewed from a distance, these slight movements affect the star's normal light spectrum, or color signature. The spectrum of a star that is moving towards the observer appears slightly shifted toward bluer (shorter) wavelengths. If the star is moving away, then its spectrum will be shifted toward redder (longer) wavelengths.

Using highly sensitive spectrographs attached to ground-based telescopes, planet hunters can track a star's spectrum, searching for periodic shifts spectral wobbles. The spectrum appears first slightly blue-shifted, and then slightly red-shifted. If the shifts are regular, repeating themselves at fixed intervals of days, months, or even years, it is almost certainly caused by a body orbiting the star, tugging it back and forth over the course of its orbit. If the body has a mass lower than about 10 times that of Jupiter (about 3,000 times the mass of Earth), then it is probably a planet. (Larger-mass objects are probably stars.)
The success of this method was made possible by the development of extremely sensitive spectrographs, which can detect even very slight movements of a star. State-of-the-art instruments such as ESPRESSO on the Very Large Telescope, EXPRES on the Lowell Observatory Discovery Channel telescope, and HARPS3 being developed for the Isaac Newton Telescope at La Palma are pushing radial-velocity sensitivity to variations as small as 10 centimeters per second.

Advantages
It's hard to argue with success. Radial velocity was the first successful method for the detection of exoplanets, and is responsible for identifying hundreds of faraway worlds. It is ideal for ground-based telescopes because (unlike for transit photometry) stars do not need to be monitored continuously. It doesn't require space-based telescopes or even the largest professional telescopes; the Isaac Newton telescope has a 2.5-meter primary mirror.
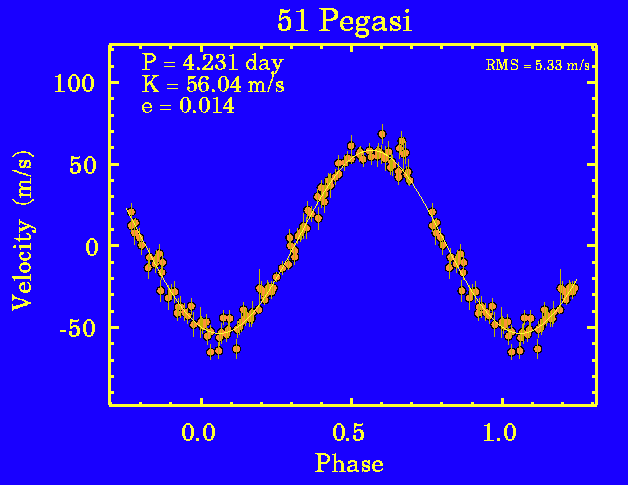
Drawbacks
It is a fundamental feature of the radial-velocity method that it cannot accurately determine the mass of a distant planet, but only provide an estimate of its minimum mass. This is a serious problem for planet hunters, because mass is the leading criterion for distinguishing between planets and small stars. Some astronomers believe that at least some of the "planets" detected by the radial-velocity method are not planets at all but very low-mass stars.
The source of this trouble with radial velocity is that the method can only detect the movement of a star towards or away from the Earth. This is not a problem if the orbital plane of the distant planetary system appears edge-on when observed from the Earth. In that case, the entire movement of the star will be towards or away from Earth, and can be detected with a sensitive spectrograph. The mass of the planet, derived from this movement, will in this case be fully accurate.
If, however, the orbital plane of a planet is face-on when observed from the Earth, the entire wobble of the star will be perpendicular to an observer's line of vision. While the star may move significantly within the orbital plane, no part of its movement will be towards or away from the Earth. No spectrum shift will be detected, and the Earth-bound observer will remain ignorant of the presence of a planet orbiting the star.
In most cases a distant planet's orbital plane is neither edge-on nor face-on when observed from Earth. Most commonly the orbital plane is tilted at some unknown angle to the line of sight. This means that a spectrograph would not detect the full movement of the star, but only that component of its wobble that moves it toward Earth or away from it. The mass of the suspected planet is directly proportional to the star's actual wobble. If only a portion of this wobble is detected, then the measured mass will be lower than the true one and provide only a minimum figure for the planet's mass.
The portion of a distant planet's mass that is detectable is determined by its orbital plane, when observed from Earth. If the angle of inclination from the face-on position is i, then the component which is in line with the Earth is given by sin(i), a number that is zero if the orbital plane is face-on to us, or one if it is edge-on. The mass of the planet as measured from Earth is therefore given by the actual planetary mass times sin(i). If i is large, i.e. the system is close to an edge-on position, then the estimated mass is close to the true one. But if i is small, and the system is, in fact, close to a face-on position, then the true mass of the "planet" is much larger than the estimate.
Only rarely do astronomers know a planetary system's true angle of inclination. This leaves open the possibility that at least some of the objects detected are too massive to be true planets.
Another drawback of the radial-velocity method is that it is most likely to find the types of planets that are the least likely to be hosts to life. Early on, most of the planets detected by spectroscopy were of a type known among scientists as hot Jupiters. These are giant planets composed mostly of gas, similar to our neighbor, Jupiter, but orbiting at dizzying speeds at a very short distance from their star. Their size, short periods, and close proximity to their star ensures that they produce the quick and relatively large stellar wobbles that are most easily detected by spectroscopy. Cooler planets orbiting further away produce more moderate wobbles in their home star, and take years to complete each orbit, factors which make them much harder to detect with spectroscopy.
But while hot Jupiters are relatively easy to find by the radial-velocity method, they are unlikely homes to any form of life as we know it. Even worse, their presence at the center of a planetary system makes it less likely that more Earthlike planets had survived in their neighborhood. In other words, while the discoveries made with spectroscopy established the presence and prevalence of planets outside our Solar System, most of the systems detected with this method are very unlikely abodes for life.
Finally, there is a practical limitation to the sensitivity of the radial-velocity method: stellar activity. Stars aren't featureless; they have brighter (hotter, hence bluer) and dimmer (cooler, hence redder) patches. As the star rotates, these patches come into and out of view. These variations in stellar color can look similar to radial-velocity signals from small, close-in planets. Our own star, the Sun, is a relatively quiet star by most standards, but its radial-velocity scatter due to spots is about 50 centimeters per second, which is 5 times bigger than the signal expected from an Earth analog. Understanding stellar activity better will be necessary to improve small-planet detections from the ground using the radial-velocity method.
This page was originally written in 2002 by staff writers for The Planetary Society. The Society most recently revised and updated it in February 2020 and thanks Emily Sandford for helpful comments.
How to Search for Exoplanets
Some methods almost sound like science fiction: Using gravity as a magnifying glass, watching stars wobble at turtle-like speeds, and searching for tiny dips in starlight.