Rosetta’s Ancient Comet
ESA Mission Unlocks the Secrets of Icy Relics
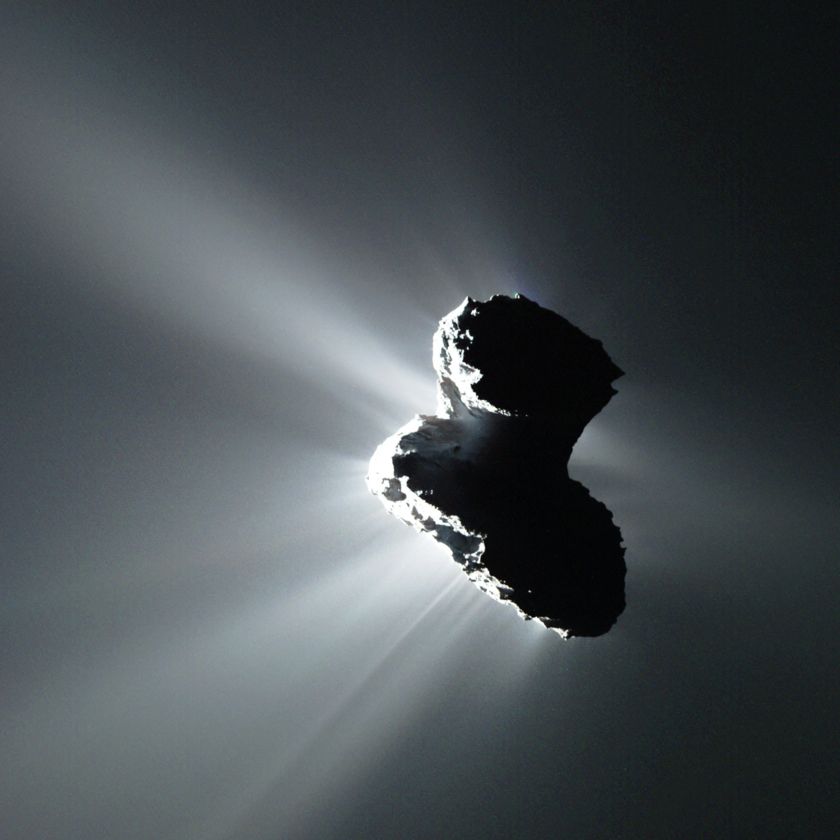
ESA/Rosetta/MPS for OSIRIS Team MPS/UPD/LAM/IAA/SSO/INTA/UPM/DASP/IDA/Jacint Roger Pérez/Emily Lakdawalla
Comet Silhouette
ESA’s Rosetta mission accompanied comet 67P/Churyumov-Gerasimenko in its orbit around the Sun from August 2014 until its landing on the comet in September 2016. The comet was most active just after perihelion in August and September 2015, its jets carrying streams of primordial dust into space.Rosetta is a European Space Agency mission with contributions from its member states and NASA. Operating such a complex mission with its 11 instruments and Philae lander is a success story in itself, but Rosetta’s greatest success is the science it delivered. Although the mission is over, the investigation of the data acquired by its instruments is bound to keep us busy for many years to come. Rosetta succeeded only because of the tremendous work and dedication of the more than 2,000 engineers, technicians, and scientists involved.

Prior comet missions have only briefly visited their targets, seeing just one moment in their evolution. Comets are dynamic worlds with dramatically varying activity. With Rosetta, we sought to visit a quiet comet and then accompany it on its path into the inner solar system, observing its evolution while approaching the Sun, passing through perihelion, and moving back into the outer solar system.
One driving question for Rosetta concerns the origin and history of comet 67P: how primordial is this cometary nucleus? How did it evolve since its formation 4.5 billion years ago? How is it evolving now? Here, we present a few selected highlights of the mission, but this is just scratching the surface and is by no means complete.

Rapidly changing nucleus
After more than 10 years of flight, Rosetta arrived at comet 67P on 6 August 2014. From earlier Hubble Space Telescope observations, we expected 67P to be more of a diamondshaped object. The peculiar bilobate shape of the comet was a big surprise. The resemblance to a rubber ducky was striking. The team quickly adopted the duck nomenclature not just for the humor of it but as a useful orientation: when somebody talked about the neck, everybody knew where to look.
Rosetta’s OSIRIS camera images of 67P revealed a nucleus with a rich variety of landforms such as steep cliffs, terraces, and circular pits, many of which exposed internal layering. The layers do not match across the two lobes, suggesting that the nucleus began as two independent objects that merged in a gentle collision. We also saw a contrast between smooth, dust-covered areas and rugged terrains.
At the time and place of arrival, well beyond 3 AU, the comet’s activity was low, releasing material at a rate of a few kilograms per second. Rosetta inspected the comet very closely, selecting a landing site and then deploying Philae late in 2014 while the low activity provided a safer environment for close approaches. Over the course of the mission, the outgassing gradually increased to several hundred kilograms per second during its peak activity some weeks after perihelion.
Rosetta and Philae’s instruments worked together to reveal the details of the structure of the comet’s surface and interior. The CONSERT experiment passed radio waves through the nucleus between the orbiter and the lander. The waves did not bounce off many internal boundaries, so the interior is probably mostly homogeneous. The surface itself is very dark, very hard, very dry (lacking much water ice), and rich in organic compounds.
High-resolution images of the southern hemisphere acquired after December 2015 revealed a clear north-south dichotomy across the nucleus’ surface. The southern hemisphere appeared rugged and more consolidated, with little dust and a flatter terrain (fewer cliffs and pits) than the northern hemisphere. The consensus is that the southern hemisphere is more representative of the comet’s interior, while the north is cloaked under a blanket of fallback dust that originated from the southern hemisphere.

The images permit us to measure the nucleus’ dimensions. The small lobe measures about 2.6 by 2.3 by 1.8 kilometers (1.6 by 1.4 by 1.1 miles), while the large lobe is about 4.1 by 3.3 by 1.8 kilometers (2.5 by 2.1 by 1.1 miles). Radio Science Investigation (RSI) measurements of Rosetta’s Doppler-shifted radio signal gave us the comet’s mass (about 1013 kilograms). Together, these numbers yield an estimated density of half that of water ice. Since the comet certainly contains denser, rocky dust material in addition to ices, it must have a high porosity of more than 70 percent. Any object moving faster than approximately 1 meter per second possesses enough energy to escape the gravitational pull of the comet. This corresponds to walking speed on Earth! During gravitationally bound orbits, the spacecraft moved even slower than that.
As Rosetta watched, the comet approached the Sun, its surface warmed up, and buried ices sublimated. The expanding gases dragged dust particles along into jets. This activity led to profound changes across the nucleus. Cliffs collapsed, fractures gaped wider, a 13-ton boulder moved, scarps appeared and/or receded, and ripples of fine-grained material formed and disappeared. Over time, the spin rate increased. Some day, that increased spin may pull the comet apart along fissures we can see in the neck.

All of these changes resulted from the comet losing mass. Comparing Doppler measurements early and late in the mission yielded an estimated mass loss of 10.5 million tons, about 0.1 percent of the comet’s total. This is equivalent to a 70-centimeter-thick (2-foot-thick) layer of material removed from the comet nucleus during each orbit. Yes, this means that comets have a finite lifetime.
Comets: Not Earth's main water source
In planetary science, elements that have a low sublimation temperature are often referred to as “volatiles.” A comet outgasses more volatiles when it’s closer to the Sun and less when it’s farther away. Like planets, comets can also have seasons. Comet 67P has pronounced seasons due to the tilt of its rotation axis with respect to the orbital plane. The northern hemisphere summer is much longer and weaker compared to the short and intense summer around perihelion in the south, and the ROSINA, MIRO, OSIRIS, and VIRTIS instruments monitored these seasonal variations.
The comet’s releases of gas and dust must originate at or near the nucleus’ surface. Yet despite all of the changes we witnessed, we had difficulty locating active areas or exposed ices on the surface of the nucleus. On rare occasions, the VIRTIS instrument detected patches of water and carbon dioxide frost on the dawn side of the nucleus.
One of the surprising results was the detection of abundant amounts of molecular oxygen (O2) by ROSINA and Alice. We saw that the abundance of oxygen correlated well with water but less well with molecular nitrogen. The relationship between oxygen and water in the comet likely dates back to their origin in the interstellar medium before the solar system formed. We also studied the isotopes of hydrogen in the comet’s water and came to a similar conclusion: the comet’s water originated in the interstellar medium.
We were surprised by the relatively large amount of heavy hydrogen (deuterium) in the comet’s water. That discovery ruled out a popular hypothesis for the origin of Earth’s water. We now know that comets of 67P’s type were not a major source of the water that became Earth’s oceans. However, noble gas measurements suggested that maybe 22 percent of Earth’s atmosphere has a cometary origin. If this is true, then organic chemicals detected by the ROSINA, COSAC, and Ptolemy mass spectrometers on both the lander and the orbiter would have also found their way to the early Earth. Organic materials that Earth potentially received from comets include a host of molecules relevant to early life, such as the simplest amino acid: glycine.

Magnetic field surprises
As Rosetta escorted the comet from 3.6 to 1.2 AU and out again, the RPC instrument suite witnessed the ever-changing comet interactions with the solar wind. When hit by solar radiation and energetic particles, the gases emanating from the nucleus lose electrons, becoming charged particles themselves. Because of their charge, they interact with the solar wind, thus forming a magnetosphere around the comet that grows as the outgassing increases. For its part, the Philae lander magnetometer detected no permanent magnetization of the nucleus, so the comet did not originate inside a strong magnetic field that left its imprint in the incorporated material.
As the comet got closer to the Sun, the comet’s magnetosphere grew and eventually blocked the solar wind from reaching the nucleus’ surface. The comet formed a “diamagnetic cavity,” a region close to the nucleus without any detectable magnetic field. Comet scientists had seen a diamagnetic cavity before during Giotto’s 14 March 1986 encounter with comet 1P/Halley. Contrary to our expectations, though, the shape of 67P’s cavity changed all the time. Rosetta’s slow velocity around the comet and the dynamic nature of the cavity’s shape meant that the boundary of the diamagnetic cavity swept across the nearly stationary spacecraft more than 600 times over the course of a few months around perihelion.
Comet dust's ancient origins
The environment of 67P proved to be quite dusty. At times, there would be so much dust near the nucleus that wayward specks would confuse Rosetta’s star trackers, and navigators had to back the spacecraft significantly away from the nucleus to avoid danger. However, Rosetta stayed as close as possible because it was at 67P to study this material. Dedicated instruments investigated the amount, composition, and shapes of dust particles around the comet. Rosetta studied dust on all scales, from meter-sized boulders seen in camera images to sub-millimeter-sized fluffy aggregates seen by the COSIMA microscope to tens of nanometers measured by the MIDAS atomic force microscope. The GIADA instrument counted particles, detecting both loosely and densely packed particles. Loosely packed agglomerations likely date from the formation processes operating in the early solar system. The densely packed particles resembled those brought back to Earth by NASA’s Stardust mission to comet 81P/Wild 2.
COSIMA measured the elemental abundances of the dust in 67P and found them to match the elemental abundance of the entire solar system, suggesting that the comet is a very primitive body dating back to solar system formation. Nearly half of the mass of 67P’s dust comprises organics. These are not small molecules; they are huge, carbonrich macromolecules similar to those found in carbonaceous chondrite meteorites. However, unlike meteorites, 67P’s dust contained silicate (rocky) minerals without any water in their crystal structures. The lack of water in 67P’s mineral particles is evidence that the comet has never been part of a larger object containing liquid water.

The comet's story
Taken together, Rosetta’s study of 67P has helped us see past the modern appearance of the comet and understand its origin. We have learned that the comet is made of primitive material, preserved almost unchanged since the comet accreted in the distant reaches of the protoplanetary disk that would eventually become our solar system. The comet contains materials that originated from a wide range of distances from the Sun and even material from the interstellar medium that predated the formation of the solar system.
The comet’s appearance today is very different from its primordial one and changes with every perihelion pass. Some comets, socalled sungrazers, do not survive their first close passage at the Sun, while others, such as 1P/Halley, may have already visited the inner solar system thousands of times. On its current orbit, 67P will return, but how many times— and in how many pieces—nobody knows. NASA is now considering a mission to bring back a sample from 67P. One day, we may return to see how much it has changed.
The Rosetta team has exerted considerable effort to make all Rosetta data publicly available. Would you like to rummage through thousands of images to find your personal favorite? Go to rosetta-osiris.eu and imagearchives.esac.esa.int.
Let’s Go Beyond The Horizon
Every success in space exploration is the result of the community of space enthusiasts, like you, who believe it is important. You can help usher in the next great era of space exploration with your gift today.
Donate TodayThe Planetary Report • June Solstice
Help advance space science and exploration! Become a member of The Planetary Society and you'll receive the full PDF and print versions of The Planetary Report.